Wissenschaft
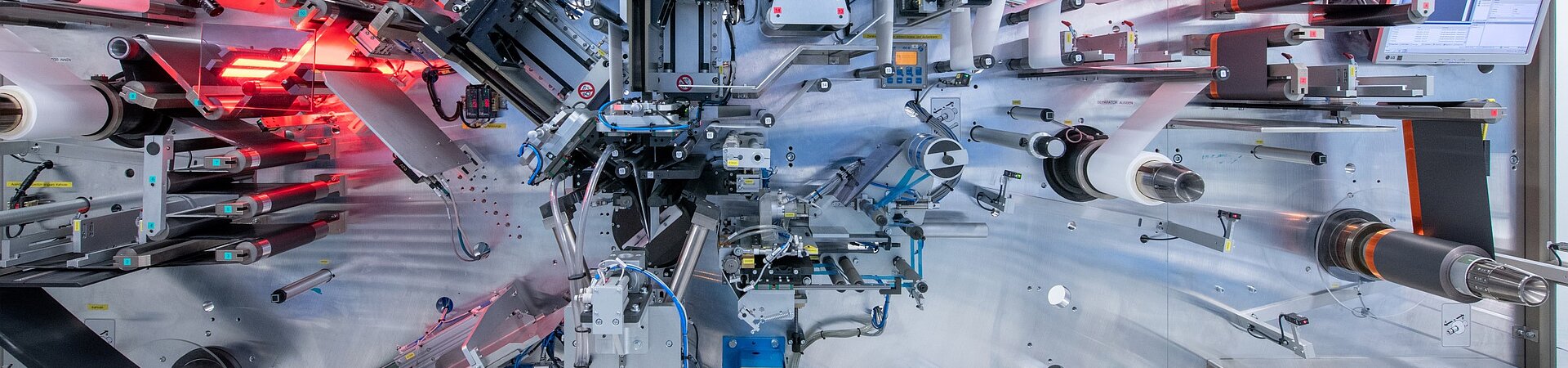
Der Exzellenzcluster POLiS erforscht neue Batteriematerialien und Technologiekonzepte für eine leistungsfähige und nachhaltige Speicherung elektrischer Energie. Ziel des Clusters ist es, Elektrodenmaterialien und Elektrolyte zu entwickeln, die nachhaltige Systeme auf der Basis von Na-, Mg-, Zn-, Ca-, Al- und Cl-Ionen ermöglichen. Größere Hürden für Entwicklung und Einsatz von Post-Lithium-Systemen und damit die Legitimation für unsere Forschung sind:
- Niedrige Mobilität von Ionen in Festkörpern und Flüssigkeiten
- Fehlen maßgeschneiderter Materialgrenzflächen mit geeigneten Ladungstransfer-Eigenschaften
- Schnelle Alterung von Aktivmaterialien und Elektrolyt
- Unzureichende Reversibilität bei Be- und Entladeprozessen
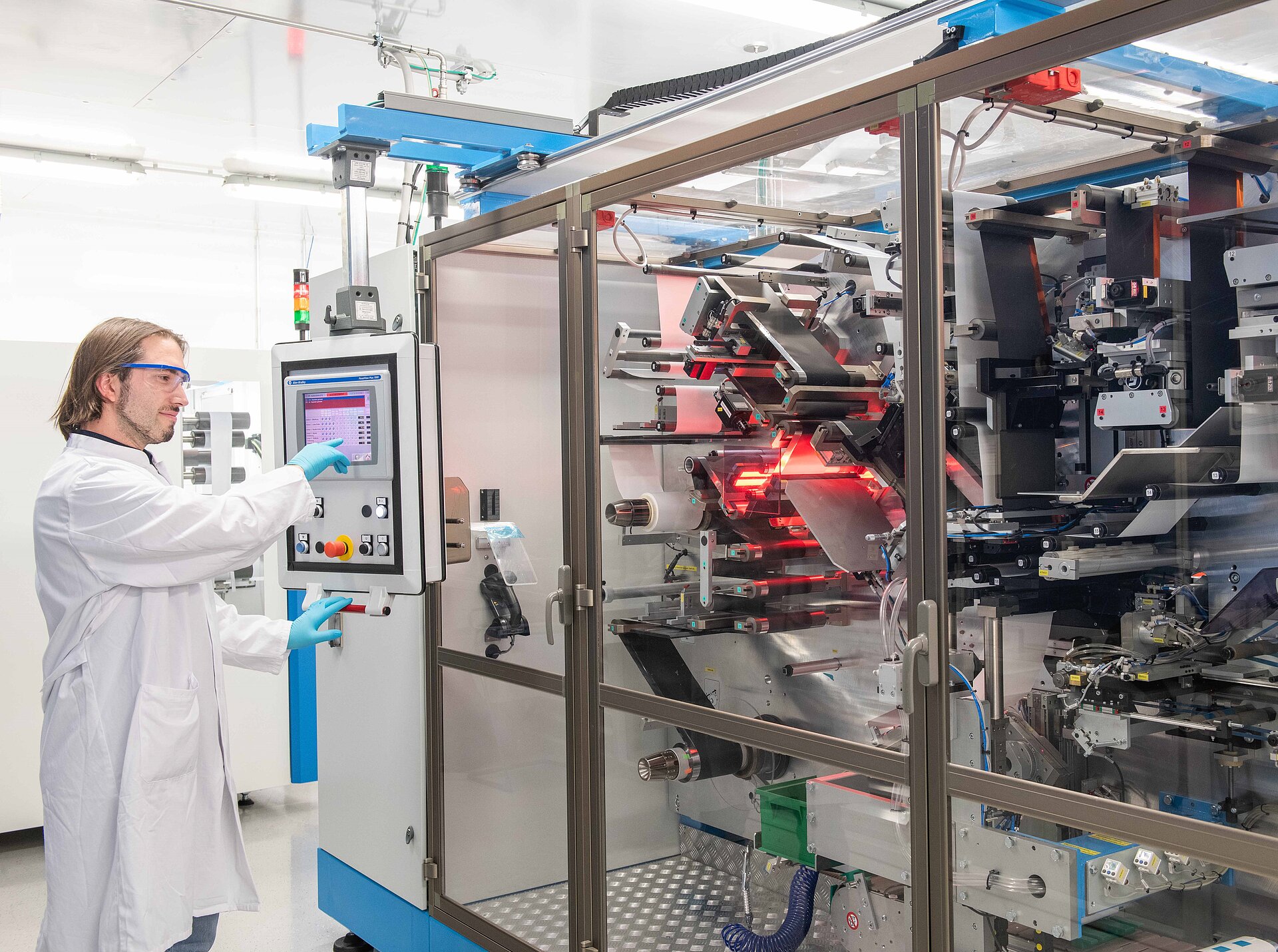
Kooperationen
Die Mitglieder des Clusters repräsentieren eine der stärksten Gruppen weltweit mit einem einzigartigen Portfolio an Kompetenzen, die für die Forschung und Entwicklung von Post-Li-Batterien relevant und notwendig sind. Die Zusammenarbeit mit nationalen und internationalen Partnern erfolgt sowohl auf der gegenseitigen Ebene direkter Kontakte zwischen den Forschern als auch über institutionalisierte Kooperationen. Ein wichtiger Aspekt ist der aktive Austausch von Personal zur Förderung wissenschaftlicher Exzellenz und gemeinsamer Projekte.
Bei Interesse an einer Kooperation mit uns, wenden Sie sich bitte an unseren Sprecher Maximilian Fichtner (+49 (0)731 50 34201, m.fichtner@kit.edu) oder direkt an einen unserer Principal Investigator, deren Kontaktdaten Sie hier finden.
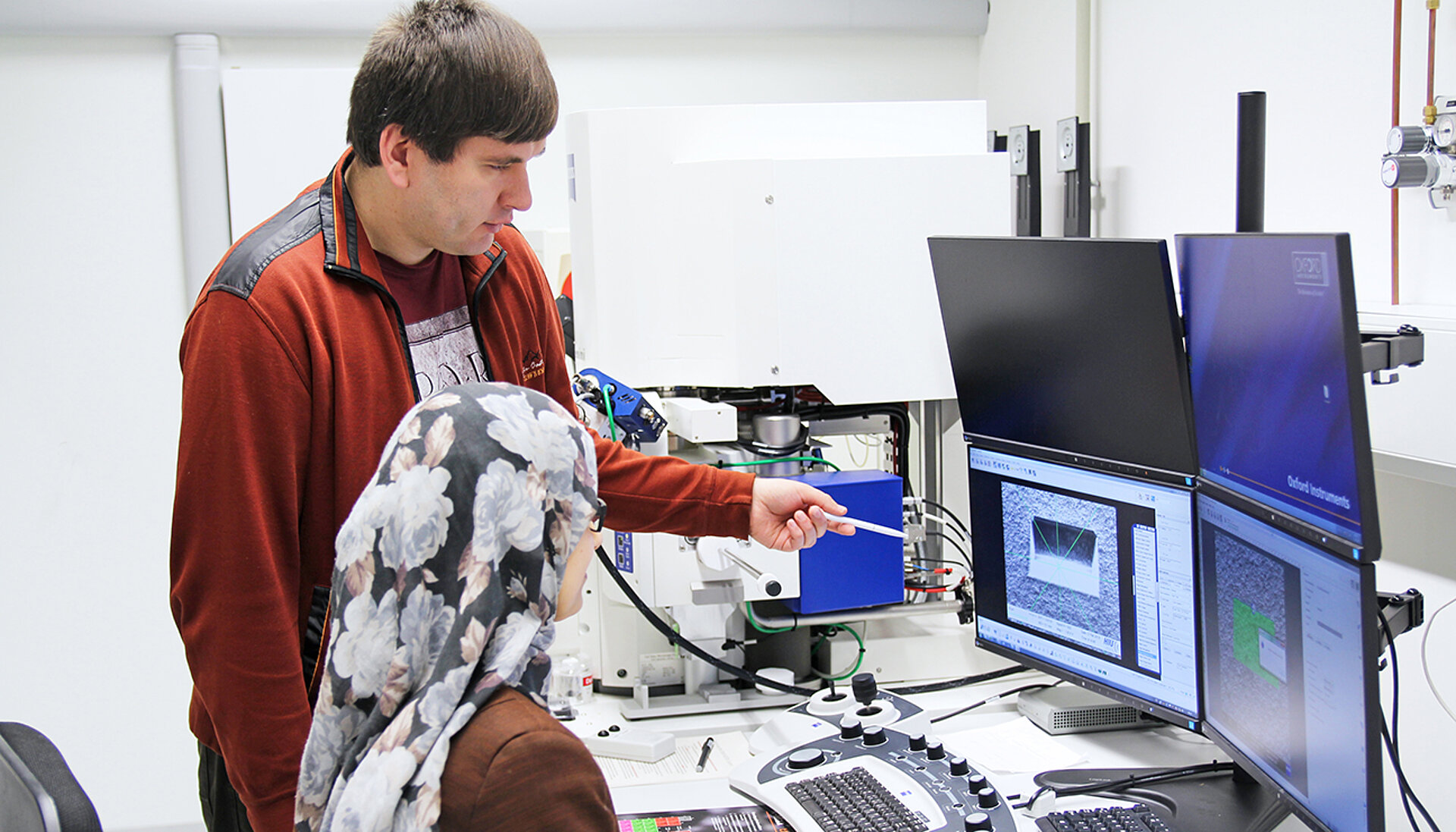
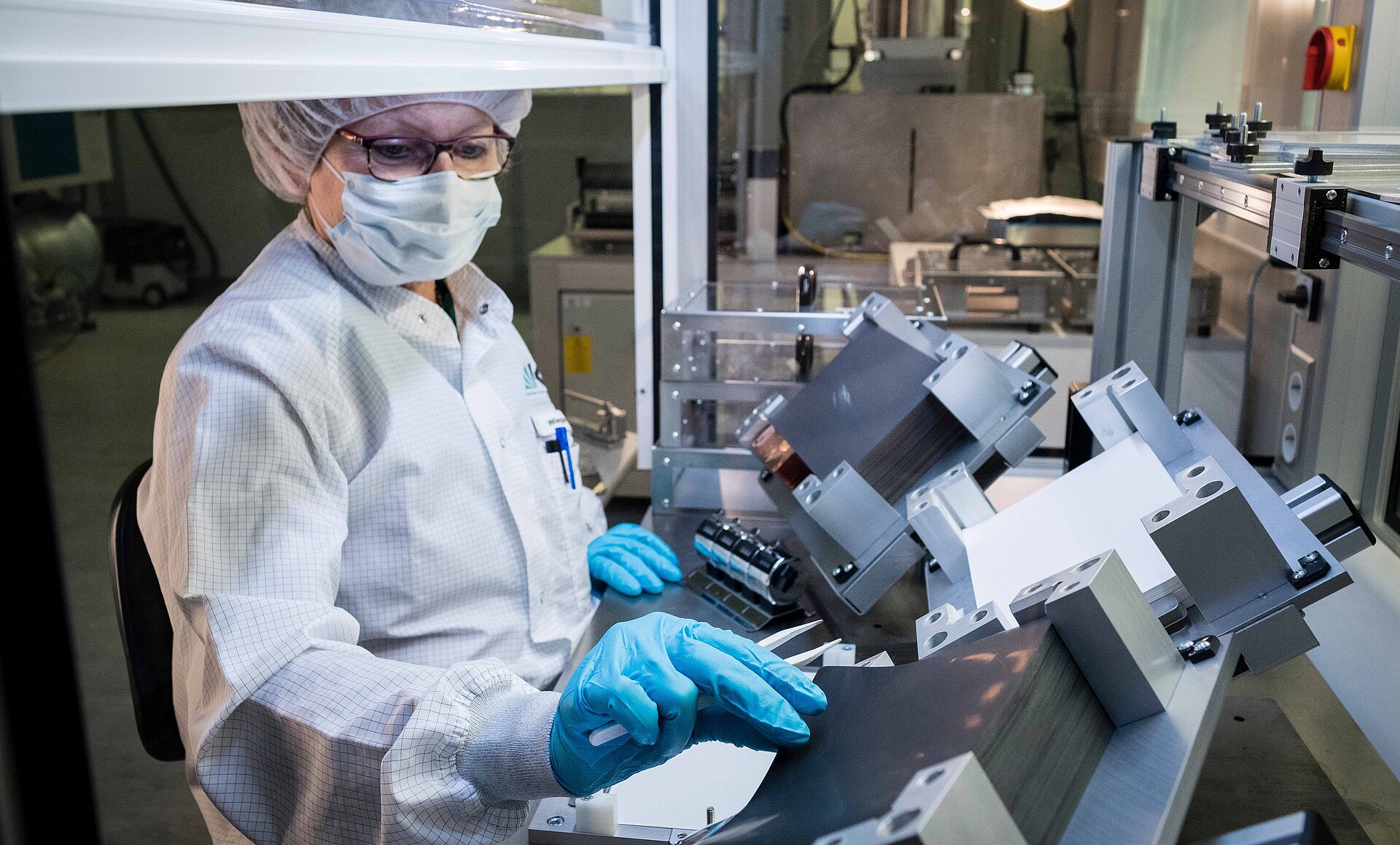
Die Arbeiten im Cluster sind in vier thematischen Säulen (Research Units) organisiert, die die zentralen Komponenten eines elektrochemischen Systems abbilden: Elektrodenmaterialien, Elektrolyte, Grenzflächen, sowie Integration und nachhaltige Zellentwicklung. Der Cluster verfolgt einen multidisziplinären Ansatz, der Nass- und Festkörperchemie, Elektrochemie, atomistische und Kontinuums-Modellierung mit Chemieingenieurwesen und Verfahrenstechnik verbindet. Die erarbeiteten Konzepte werden auf Batterie-Vollzellen übertragen, um sie auf Leistungsfähigkeit, Nachhaltigkeit und Sicherheit zu überprüfen. Es gibt eine rasch steigende Nachfrage für zukünftige Batterien, die Folgendes umfassen und unsere Forschung definieren:
- Nachhaltige Materialien und Herstellungsverfahren
- Erhöhte volumetrische/gravimetrische Energiedichte
- Eigensichere Konstruktion
- Lange Betriebs- und Haltbarkeitsdauer
- Niedriger Preis pro kWh
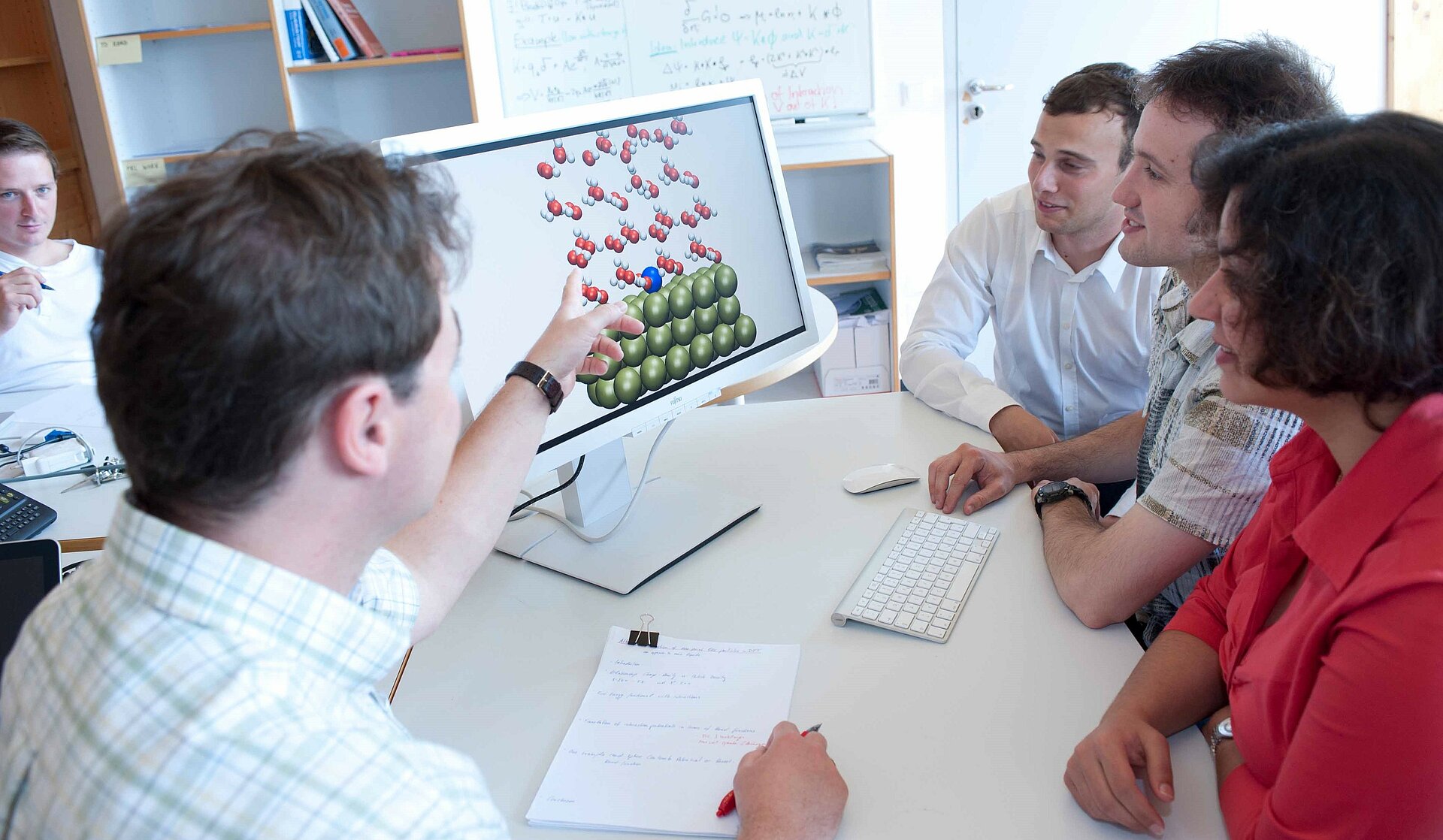
Karriereförderung von Nachwuchsforscher*innen
Die Karriereaussichten von Nachwuchsforscher*innen bei POLiS sind aufgrund der hohen wissenschaftlichen und industriellen Relevanz des Forschungsprogramms hervorragend, was sich in einer hohen Nachfrage nach gut ausgebildetem Fachpersonal in den relevanten Branchen, etwa bei Batteriematerialzulieferern oder in der Automobil- und Elektronikindustrie, zeigt.
Der Cluster bietet in Zusammenarbeit mit der Graduiertenschule (GS-EES) ein strukturiertes Qualifizierungsprogramm an. Weitere Informationen finden Sie hier. Wir freuen uns auf Ihre aussagekräftige Initiativbewerbung, die Sie bitte an den entsprechenden Principal Investigator richten, der sich mit dem für Sie interessanten Forschungsgebiet beschäftigt.
Struktur
Forschungsthemen
Research Unit A (Electrode Materials)
Electrode materials define and limit the amount of energy that can be stored per weight and volume of a battery. Our goal is to identify, synthesize and test new high-capacity post-lithium storage materials and thereby gain an even deeper understanding of their functionality.
Spokesperson
Work Package A.1 – Monovalent ions
This work package is essential for reaching the overall goal to deliver large-format SIBs with high performance and to provide suitable materials and electrodes for other work packages. The focus is on layered oxides for positive electrode materials and on carbon-based negative electrodes, investigated in a comprehensive interdisciplinary approach.
Work Package A.2 – Multivalent Ions
This WP focuses on the development of electrode materials for battery chemistries based on multivalent ions (Al, Ca, Mg). The main activities aim at designing and synthesizing new or improved electrode materials. Scope of this WP is to the shed light on transport and kinetics descriptors of multivalent ions into solids by combining experimental and theoretical approaches in order to rationalize the further improvement of the proposed materials.
Work Package A.3 – Anionic Systems
The work package ‘Anionic Systems’ aims to synthesize novel electrode materials and investigate them both experimentally and theoretically. Also it will provide an overview about the interphase formation mechanism in CIB’s. Overall, this work package bridges missing links between electrode, electrolyte in chloride ion batteries and could pave way for the acceleration of research efforts on anionic batteries across the research community.
Research Unit B (Electrolyte)
Electrolytes enable the transport of electrically charged particles (ions) between the two electrodes of a battery. The main objective is the identification, synthesis and testing of new, stable and highly efficient liquid or solid transfer systems for post-lithium-ions.
Spokespersons
Work Package B.1 – Liquid Electrolytes
Any battery needs an appropriate electrolyte. Thus, the electrolyte is a key component as it connects both electrodes and allows an ionic and electronic communication between both. While at the electrodes ions are either intercalated in an existing structure or converted electrochemically by charge transfer processes, the development of suitable electrolytes faces various challenges, including stability, integrity, SEI formation processes, as well as charge and ion conductivity. This work package aims at the development of liquid electrolytes with high ionic conductivities and chemical and electrochemical stability. To tackle this challenge, different activities have been identified with the goal to synthesize and test new liquid electrolytes for mono- as well as multi-valent ions, to elucidate the mechanisms of ionic transport in these systems and to deduce strategies for better electrolytes.
Work Package B.2 – Solid Electrolytes
Solid-state batteries are considered as the ultimate solution of safety and stability issues, and their exploration and development will be a long-term enterprise. As necessary materials´ basis, solid electrolytes with a combination of various properties need to be developed, of which the high ionic conductivity (combined with low electronic conductivity), the (kinetic) stability in contact with electrode materials and their mechanical properties are important “key performance indicators”. This WP aims to be the cental WP of POLIS to explore, understand, characterize and test solid electrolytes for post-lithium systems.
Research Unit C (Interfaces)
We aim to understand the formation and nature of interfaces that form within a post-Li battery - e.g. at the contact between electrode and liquid or solid electrolyte. Their microstructure and chemical composition have a decisive influence on the interface processes within the battery and thus determine, among other things, its performance and longevity.
Spokespersons
Work Package C.1 – Structure, Function and Morphology at Metal Electrodes
Metal deposition/dissolution and SEI formation at different metal electrodes will be investigated by various experimental methods in order to obtain detailed mechanistic understanding of the underlying processes, reaching from the charge transfer processes across the interphase to structure formation and morphological changes, SEI formation and side processes like electrolyte decomposition. The experimental studies will be conducted in strong collaboration with theoretical investigations spanning from atomistic investigations of elementary processes to MD simulations and modelling on different length scales. The work program will focus on exemplary studies on a limited number of systems, e.g., Na, Mg and Al deposition from prototypical electrolytes to ensure comparability of the results obtained from different methods.
Work Package C.2 – Structure, Function and Morphology at Carbon Electrodes
This work package aims to get a holistic knowledge about ongoing reactions and kinetics at carbon anodes. To get maximum knowledge gain, we focus in our work on commonly defined carbon systems. The whole available POLiS expertise will be used to clarify ongoing atomic processes within the electrode as well as on the electrode surface considering SEI formation due to electrolyte decomposition during cycling. Only through a complete description and holistic understanding of the electrode behavior, it is possible to improve the electrodes in terms of performance and long cycling stability. A targeted added value is also developed from the methodology of how the individual analysis techniques can be brought together synergistically in order to obtain new, deeper, comprehensive insights into SEI on electrode surfaces. The knowledge gained from the selected model system (SIB) can be transferred to other, new battery systems inside and outside the POLiS cluster and can make a targeted contribution to improving the interface kinetics of next-generation batteries.
Work Package C.3 – Positive Electrodes
For positive electrodes with both solid and liquid electrolytes, the transfer processes at interfaces are decisive for the overall performance of the battery. Interfacial reactions at equilibrium and at various operation states, ion migration kinetics, the role of grain and phase boundaries and dendrite formation are all important aspects that require more indepth investigations to develop a better description of these processes and an understanding of their role in battery performance. Furthermore, interfacial processes are key to understand CEI formation and material degradation. The aim is to use this knowledge in order to optimize kinetics and to suppress degradation processes.
Research Unit D (Integration and Sustainable Cell Engineering)
Up-scaling of materials synthesis and cell construction, studies on the production capability of new technical approaches, assessment of cell safety and life cycle analyses of new technologies will pave the way for technology transfer. Central research data management and the development of automated data analysis tools will flank all work in the Cluster, set standards for the handling of research data and enable integrative, cross-research field data analysis, which we hope will lead to new insights in battery research.
Spokespersons
Work Package D.1 – Cell Design & Production
It intends to develop complete processing chains for post-Li systems including the scale-up of materials production, making electrode and cell manufacturing more sustainable and reliable, and the development of material-process-models to generate a deep understanding of the material behavior during processing. WP D1 is therefore, a very interdisciplinary research unit, where chemists, material scientists and process engineers tackle the complex tasks to enable the best performance from new materials in cells by developing the optimal conditions for the material, such as structure of the material, material integrity within the electrode, and overall electrode design, including its further processing up to optimal cell assembly. Another focus is on sustainable electrode and battery production through tailored manufacturing strategies depending on the material, components and composition. In the current phase, the results obtained in the material and process development activities to date are to be combined in order to lead to high-performance POLiS cells with high capacity.
Work Package D.2 – Demonstrator Cell
This WP addresses projects where the most promising post-lithium chemistries developed in POLiS (either material synthesis or its processing) are scaled up to the pouch cell level. On the one hand, a prototype lab-pouch cell serves as a proof of principle for poorly developed cell chemistries (e.g., Aluminum). On the other hand, full industrial-type pouch cells are used to demonstrate the performance of highly developed materials (e.g. Sodium). Na-, Mg- and Al-based chemistries will be studied in the full cell configuration to push each chemistry towards the next developmental step. The results from WP D2 have a great impact on POLiS as the POLiS demonstrator cell’s performance reflects the success of a complex and interdisciplinary research chain throughout all research units. The success of a cell chemistry as a demonstrator on pouch-cell level is the ultimate proof that all aspects of material, interphase, electrolyte material processing and engineering have successfully come together.
Work Package D.3 – Safety & Sustainability
Upscaling of both cathode materials as pouch cells are ongoing in cooperation with BaTec (IAM-ESS) and in similar manner, safety analysis on pouch cell level shall be performed and their heat generation during operation (under normal condition) shall be measured using Heat Flux sensors followed by thermal abuse tests using the Heat-Wait-Seek (HWS) method in the ARC. The sparation of reversible and irreversible heat as well as out gases anaylsis during cell-formation step and during thermal abuse will be also considered.
Also the sustainability of new materials and cell will be analysed in a prospective manner. The focus will be on Na- and Mg-Batteries and fluid and solid electrolyte and the prospective assessment of upscaled production process (including printing production process).The kind of analyses helps to improve the systems under development and prevent mismatch regrading the expectations of stakeholders and society regarding sustainability.
Research Unit X (Cross-sectional Topics)
In this research unit, innovative topics such as potassium batteries, organic redox systems, AI-supported virtual simulations or degradation are investigated. For this purpose, the competences from all other units are combined.
Spokespersons
Work Package X.1 – High-Energy Potassium Batteries
The overarching goal of this work package is to build high-energy potassium-ion batteries by combining the competences of the clusters’ four original research units to one coherent topical work plan. In recent years, the field of potassium batteries greatly benefitted from the broad knowledge base available for the related monovalent battery systems, namely lithium- and sodium-ion batteries, and accelerated the developments in this field considerably. However, the high reactivity of potassium or potassium storing active materials favours different electrolyte degradation pathways, leading to significant changes of the SEI properties and hence cycle life. It is therefore necessary to detach from established concepts of previous technologies and provide new, system-specific strategies that enable mature potassium-ion systems. Providing a holistic approach, ranging from liquid to solid electrolytes over new electrode material developments to in-depth characterization on material and cell level is a globally unique measure of the work package.
Work Package X.2 Organic Redox Systems
The aim of the WP “Organic Redox Systems” is to purposefully design, synthesize and test new functional organic materials for Post-Li storage. For this purpose, the activities in the WP will focus on two subprojects.
Project I on porphyrin based electrodes and project II on organic electrodes based on quinones and other functional groups. Project I is a further development of the already established organic electrode material of [5,15-bis-(ethynyl)-10,20-diphenylporphinato]copper(II) (CuDEPP), which has a capacity comparable to an LFP cathode, while its rate capability is comparable to that of a supercapacitor (50C). To improve the performance and stability of the post-Li cells with metal porphyrins, we will investigate the influence of different metal centers as well as side groups and introducing of novel functionalities.
In the second project, novel high capacity and high voltage n- and p-type electrodes will be synthesized. Special attention will be paid on designing organic redox materials with limited solubility in the electrolytes. The developed material will be electrochemically analyzed. Upon optimizing the electrode and selecting appropriate electrolyte, it will be tested for post-Li storage (mainly Na-, Mg-, and Ca systems). We anticipate that the work will provide fundamental understanding of the storage mechanism in organic redox systems as well as the establishment of structure-property-performance relationship in the organic electrodes which could be used toward rational and target-oriented development of organic electrodes for post-Li storage.
Work Package X.3 Degradation
This work package is of an overarching nature in POLiS, as degradation processes can reduce the performance and lifetime of all components of post-Li batteries. The majority of projects in this WP follow a rational approach to prevent or suppress degradation processes. By a careful characterization of the degradation, identification of degradation products and a thorough analysis of the origins of these degradation processes, strategies to suppress degradation shall be developed. To achieve this goal, degradation shall be studied both experimentally and theoretically on several length and time scales.
In a second approach, pulsed charging protocols shall be developed from combining a series of experimental techniques with simulation tools that allow for ultra fast charging without shortening the lifetime of post-Li batteries. From an analysis of the optimized charging protocols, also an better understanding of degradation processes shall be developed. These two complimentary approaches will together identify how degradation processes in post-Li batteries can be avoided, so that the lifetime of the batteries will be extended.
Work Package X.4 AI Enhanced Virtual Simulation Chain
Adressing the challenges in developing efficient, sustainable and robust Post-Lithium batteries requires a strongly coupled simulation approach comprising all length and times scales and a systematic coupling to data based simulation techniques. Within the POLiS clusters all necessary simulation competences are available to address this key challenge for accelerating the development of Post-Lithium batteries.
Objectives of the work package are, first, to gain an understanding of the factors underlying these structure and processes in Post-Lithium batteries on all involved length and time scales. Second, by using a vertical multi scale approach with validated algorithms and description to compute parameters that are transferred from one level to the next, the reliability of the theoretical and numerical results shall be improved and coupling to data driven simulation techniques will be developed.
Work Package X.5 Advanced Integrated Data Analysis & Descriptors
The work package addresses the advanced data analysis techniques, high-throughput simulations and the concept of deriving structure-property relationships by descriptors for post-Li battery systems. While the analysis techniques will be used to bridge results from experiments, theoreticians and simulations by data exchange, high-throughput simulations will serve as concept for parameter studies on different scales to create digital twins. With descriptors, correlations between fundamental materials properties and desired or undesired functional properties of the materials are extracted. In order to improve the efficiency of post-Li battery research within the work package as well as within the whole Cluster Kadi4Mat is strongly promoted as the research data infrastructure. Consequently, Kadi4Mat is the base for creating reusable workflows for data analysis, simulations and descriptors.
Publikationen
Publikationsliste
-
2025A Bifunctional Iron‐Nickel Oxygen Reduction/Oxygen Evolution Catalyst for High‐Performance Rechargeable Zinc–Air Batteries
Chen, Z.; Cheng, W.; Cao, K.; Jin, M.; Rahali, S.; Chala, S. A.; Ebrahimi, E.; Ma, N.; Liu, R.; Lakshmanan, K.; Chang, C.-Y.; Cheung, C.-C.; Luo, H.; Wang, Y.; Hwang, B. J.; Streb, C.
2025. Small, 21 (3). doi:10.1002/smll.202409161Role of Desolvation upon the Sodiation of Hard Carbon in Sodium-Ion Batteries: A Microcalorimetric Study of the Sodiation Entropy
Derr, L.; Lang, M.; Palanisamy, K.; Kranz, C.; Schuster, R.
2025. The Journal of Physical Chemistry C, 129 (8), 4025–4031. doi:10.1021/acs.jpcc.4c08586Calcium Chemistry as A New Member of Post‐Lithium Battery Family: What Can We Learn from Sodium and Magnesium Systems
Li, Z.; Cui, S.; Häcker, J.; Nojabaee, M.; Fichtner, M.; Cui, G.; Zhao-Karger, Z.
2025. Angewandte Chemie - International Edition, 64 (5), Art.-Nr.: e202415942. doi:10.1002/anie.202415942Simultaneously improving sodium ionic conductivity and dendrite behavior of NaSICON ceramics by grain-boundary modification
Liu, L.; Ma, Q.; Zhou, X.; Ding, Z.; Grüner, D.; Kübel, C.; Tietz, F.
2025. Journal of Power Sources, 626, 235773. doi:10.1016/j.jpowsour.2024.235773How reference electrodes improve our understanding of degradation processes in half and full cell potassium-ion battery setups
Panasenko, I.; Bäuerle, M.; Jeschull, F.
2025. Electrochimica Acta, 513, 145551. doi:10.1016/j.electacta.2024.145551Synthesis, Structural Analysis, and Degradation Behavior of Potassium Tin Chloride as Chloride‐Ion Batteries Conversion Electrode Material
Panja, S.; Miao, Y.; Döhn, J.; Choi, J.; Fleischmann, S.; Guddehalli Chandrappa, S.; Diemant, T.; Groß, A.; Karkera, G.; Fichtner, M.
2025. Advanced Functional Materials, 35 (3), Art.-Nr.: 2413489. doi:10.1002/adfm.202413489Redox Self‐Equilibration in Molecular Vanadium Oxide Mixtures Enables Multi‐Electron Storage
Remmers, M.; Mashtakov, B.; Repp, S.; Rein, A. S. J.; Wang, K.; Anjass, M.; Chen, Z.; Carrella, L. M.; Rentschler, E.; Streb, C.
2025. Angewandte Chemie International Edition, 64 (2). doi:10.1002/anie.202418864Exploring the possibility of aluminum plating/stripping from a non‐corrosive Al(OTf)3‐based electrolyte
Talari, M.; Sarapulova, A.; Zemlyanushin, E.; Sabi, N.; Hofmann, A.; Trouillet, V.; Dsoke, S.
2025. Batteries & Supercaps, 8 (1), Art.-Nr.: 202400317. doi:10.1002/batt.202400317Dissolution of molybdenum current collector as Crucial and Undesired process in aluminum batteries
Zemlyanushin, E.; Schwarz, B.; Dsoke, S.
2025. Journal of Power Sources, 633, 236458. doi:10.1016/j.jpowsour.2025.236458 -
2024Pd‐Catalyzed Oxidative C−H Arylation of (Poly)fluoroarenes with Aryl Pinacol Boronates and Experimental and Theoretical Studies of its Reaction Mechanism
Budiman, Y. P.; Putra, M. H.; Ramadhan, M. R.; Hannifah, R.; Luz, C.; Ghafara, I. Z.; Rustaman, R.; Ernawati, E. E.; Mayanti, T.; Groß, A.; Radius, U.; Marder, T. B.
2024. Chemistry – An Asian Journal, 19 (8), Art.-Nr. e202400094. doi:10.1002/asia.202400094π‐Conjugated Metal Free Porphyrin as Organic Cathode for Aluminum Batteries
Chowdhury, S.; Sabi, N.; Rojano, R. C.; Le Breton, N.; Boudalis, A. K.; Klayatskaya, S.; Dsoke, S.; Ruben, M.
2024. Batteries & Supercaps, 7 (4), Art.-Nr.: e202300285. doi:10.1002/batt.202300285Influence of Electrode Structuring Techniques on the Performance of All‐Solid‐State Batteries
Clausnitzer, M.; Danner, T.; Prifling, B.; Neumann, M.; Schmidt, V.; Latz, A.
2024. Batteries & Supercaps, 7 (4), Art.-Nr.: e202300522. doi:10.1002/batt.202300522Elucidating Gas Evolution of Prussian White Cathodes for Sodium‐ion Battery Application: The Effect of Electrolyte and Moisture
Dreyer, S. L.; Maddar, F. M.; Kondrakov, A.; Janek, J.; Hasa, I.; Brezesinski, T.
2024. Batteries & Supercaps, 7 (4), e202300595. doi:10.1002/batt.202300595Systematic review of scale-up methods for prospective life cycle assessment of emerging technologies
Erakca, M.; Baumann, M.; Helbig, C.; Weil, M.
2024. Journal of Cleaner Production, 451, 142161. doi:10.1016/j.jclepro.2024.142161MgO coated P -Na Mn Ni O layered oxide cathode for Na-Ion batteries
Gauckler, C.; Kucinskis, G.; Pfeiffer, L. F.; Abdellatif, A. A.; Tang, Y.; Kübel, C.; Maroni, F.; Gong, R.; Wohlfahrt-Mehrens, M.; Axmann, P.; Marinaro, M.
2024. Journal of Power Sources Advances, 25, 100135. doi:10.1016/j.powera.2024.100135A Stable High-Potential Na₇V₄(P₂O₇)₄(PO₄) Cathode for Sodium-Ion Batteries Developed from a Water-Based Slurry
Gong, R.; Maroni, F.; Marinaro, M.
2024. Journal of The Electrochemical Society, 171 (4), 040508. doi:10.1149/1945-7111/ad36e8In Situ Monitoring of the Al(110)‐[EMImCl] : AlCl 3 Interface by Reflection Anisotropy Spectroscopy
Guidat, M.; Rahide, F.; Löw, M.; Kim, J.; Ehrenberg, H.; Dsoke, S.; May, M. M.
2024. Batteries & Supercaps, 7 (1), Art.-Nr.: e202300394. doi:10.1002/batt.202300394Detection of Charge‐Neutral Near‐Equilibrium Processes at Na‐Metal Electrodes by Electrochemical Microcalorimetry
Karcher, F.; Uhl, M.; Geng, T.; Jacob, T.; Schuster, R.
2024. Advanced Energy Materials, 14 (3), Art.-Nr.: 2302241. doi:10.1002/aenm.202302241Impact of Nano‐sized Inorganic Fillers on PEO‐based Electrolytes for Potassium Batteries
Khudyshkina, A. D.; Rauska, U.-C.; Butzelaar, A. J.; Hoffmann, M.; Wilhelm, M.; Theato, P.; Jeschull, F.
2024. Batteries and Supercaps, 7 (1), Art.-Nr.: e202300404. doi:10.1002/batt.202300404Studies on 3D printing of Na3Zr2Si2PO12 ceramic solid electrolyte through Fused Filament Fabrication
Kutlu, A. C.; Nötzel, D.; Hofmann, A.; Ziebert, C.; Seifert, H. J.; Mohsin, I. U.
2024. Electrochimica Acta, 503, Art.-Nr.: 144881. doi:10.1016/j.electacta.2024.144881Cover Feature: 3D Printing of Na1.3Al0.3Ti1.7(PO4)3 Solid Electrolyte via Fused Filament Fabrication for All-Solid-State Sodium-Ion Batteries (Batteries & Supercaps 1/2024)
Kutlu, A. C.; Nötzel, D.; Ziebert, C.; Seifert, H. J.; Ul Mohsin, I.
2024. Batteries & Supercaps, 7 (1), Art.-Nr.: e202300577. doi:10.1002/batt.2023005773D Printing of Na Al Ti (PO ) Solid Electrolyte via Fused Filament Fabrication for All‐Solid‐State Sodium‐Ion Batteries
Kutlu, A. C.; Nötzel, D.; Ziebert, C.; Seifert, H. J.; Mohsin, I. U.
2024. Batteries & Supercaps, 7 (1), e202300357. doi:10.1002/batt.202300357The relevance of structural variability in the time-domain for computational reflection anisotropy spectroscopy at solid–liquid interfaces
Leist, J.; Kim, J.; Euchner, H.; May, M. M.
2024. Journal of Physics: Condensed Matter, 36 (18), Art.-Nr.: 185002. doi:10.1088/1361-648X/ad215bMagnesium and Aluminum in Contact with Liquid Battery Electrolytes: Ion Transport through Interphases and in the Bulk
Löw, M.; Grill, J.; May, M. M.; Popovic-Neuber, J.
2024. ACS Materials Letters, 6 (11), 5120–5127. doi:10.1021/acsmaterialslett.4c01589Nucleation Mechanisms of Electrodeposited Magnesium on Metal Substrates
Löw, M.; Maroni, F.; Zaubitzer, S.; Dongmo, S.; Marinaro, M.
2024. Batteries & Supercaps, 7 (11). doi:10.1002/batt.202400250Exploring the reactivity of Na₃V₂(PO4)₃/C and hard carbon electrodes in sodium-ion batteries at various charge states
Mohsin, I. U.; Hofmann, A.; Ziebert, C.
2024. Electrochimica Acta, 487, Article no: 144197. doi:10.1016/j.electacta.2024.144197Stochastic 3D Modeling of Nanostructured NVP/C Active Material Particles for Sodium‐Ion Batteries
Neumann, M.; Philipp, T.; Häringer, M.; Neusser, G.; Binder, J. R.; Kranz, C.
2024. Batteries & Supercaps, 7 (4). doi:10.1002/batt.202300409Deposition of Sodium Metal at the Copper‐NaSICON Interface for Reservoir‐Free Solid‐State Sodium Batteries
Ortmann, T.; Fuchs, T.; Eckhardt, J. K.; Ding, Z.; Ma, Q.; Tietz, F.; Kübel, C.; Rohnke, M.; Janek, J.
2024. Advanced Energy Materials, 14 (15), Art.-Nr.: 2302729. doi:10.1002/aenm.202302729Microscopic and Spectroscopic Analysis of the Solid Electrolyte Interphase at Hard Carbon Composite Anodes in 1 M NaPF /Diglyme
Palanisamy, K.; Daboss, S.; Romer, J.; Schäfer, D.; Rohnke, M.; Flowers, J. K.; Fuchs, S.; Stein, H. S.; Fichtner, M.; Kranz, C.
2024. Batteries and Supercaps, Art.Nr.: e202300482. doi:10.1002/batt.202300482Spray‐coated Hard Carbon Composite Anodes for Sodium‐Ion Insertion
Palanisamy, K.; Daboss, S.; Schäfer, D.; Rohnke, M.; Derr, L.; Lang, M.; Schuster, R.; Kranz, C.
2024. Batteries and Supercaps, 7 (1), Art.-Nr.: e202300402. doi:10.1002/batt.202300402Modification of Al Surface via Acidic Treatment and its Impact on Plating and Stripping
Rahide, F.; Palanisamy, K.; Flowers, J. K.; Hao, J.; Stein, H. S.; Kranz, C.; Ehrenberg, H.; Dsoke, S.
2024. ChemSusChem, 17 (5), Art.Nr.: e202301142. doi:10.1002/cssc.202301142Improving rechargeable magnesium batteries through dual cation co-intercalation strategy
Roy, A.; Sotoudeh, M.; Dinda, S.; Tang, Y.; Kübel, C.; Groß, A.; Zhao-Karger, Z.; Fichtner, M.; Li, Z.
2024. Nature Communications, 15 (1), Art.-Nr.: 492. doi:10.1038/s41467-023-44495-2PFAS-Free Energy Storage: Investigating Alternatives for Lithium-Ion Batteries
Savvidou, E. K.; Rensmo, A.; Benskin, J. P.; Schellenberger, S.; Hu, X.; Weil, M.; Cousins, I. T.
2024. Environmental Science & Technology, 58 (50), 21908–21917. doi:10.1021/acs.est.4c06083Multiscale Investigation of Sodium‐Ion Battery Anodes: Analytical Techniques and Applications
Schäfer, D.; Hankins, K.; Allion, M.; Krewer, U.; Karcher, F.; Derr, L.; Schuster, R.; Maibach, J.; Mück, S.; Kramer, D.; Mönig, R.; Jeschull, F.; Daboss, S.; Philipp, T.; Neusser, G.; Romer, J.; Palanisamy, K.; Kranz, C.; Buchner, F.; Behm, R. J.; Ahmadian, A.; Kübel, C.; Mohammad, I.; Samoson, A.; Witter, R.; Smarsly, B.; Rohnke, M.
2024. Advanced Energy Materials, 14 (15), Art.-Nr.: 2302830. doi:10.1002/aenm.202302830Conjugated Polyimidazole Nanoparticles as Biodegradable Electrode Materials for Organic Batteries
Schuster, P. A.; Uhl, M.; Kissmann, A.-K.; Jansen, F.; Geng, T.; Ceblin, M. U.; Spiewok, S.; Rosenau, F.; Jacob, T.; Kuehne, A. J. C.
2024. Advanced Electronic Materials, 10 (4), Art.-Nr.: 2300464. doi:10.1002/aelm.202300464Magnetic Single‐Ion Anisotropy and Curie‐Weiss Behaviour of Mg₃V₄(PO₄)₆
Schwarz, B. C.; Fu, Q.
2024. European Journal of Inorganic Chemistry, 27 (18), e202400162. doi:10.1002/ejic.202400162Ion Mobility in Crystalline Battery Materials
Sotoudeh, M.; Baumgart, S.; Dillenz, M.; Döhn, J.; Forster-Tonigold, K.; Helmbrecht, K.; Stottmeister, D.; Groß, A.
2024. Advanced Energy Materials, 14 (4), Art.Nr.: 2302550. doi:10.1002/aenm.202302550From Powder to Pouch Cell: Setting up a Sodium‐Ion Battery Reference System Based on Na₃V₂(PO₄)₃/C and Hard Carbon
Stüble, P.; Müller, C.; Bohn, N.; Müller, M.; Hofmann, A.; Akçay, T.; Klemens, J.; Koeppe, A.; Kolli, S.; Rajagopal, D.; Geßwein, H.; Schabel, W.; Scharfer, P.; Selzer, M.; Binder, J. R.; Smith, A.
2024. Batteries & Supercaps, 7 (12), e202400406. doi:10.1002/batt.202400406Enabling Long‐term Cycling Stability of Na₃V₂(PO₄)₃ /C vs . Hard Carbon Full‐cells
Stüble, P.; Müller, C.; Klemens, J.; Scharfer, P.; Schabel, W.; Häringer, M.; Binder, J. R.; Hofmann, A.; Smith, A.
2024. Batteries and Supercaps, 7 (2), Art.-Nr. e202300375. doi:10.1002/batt.202300375Recent developments and future prospects of magnesium–sulfur batteries
Wang, L.; Riedel, S.; Drews, J.; Zhao-Karger, Z.
2024. Frontiers in Batteries and Electrochemistry, 3. doi:10.3389/fbael.2024.1358199Challenges and Progress in Anode‐Electrolyte Interfaces for Rechargeable Divalent Metal Batteries
Wang, L.; Riedel, S.; Zhao-Karger, Z.
2024. Advanced Energy Materials, 14 (38), Art.-Nr.: 2402157. doi:10.1002/aenm.202402157Exploration of the Lithium Storage Mechanism in Monoclinic Nb O as a Function of the Degree of Lithiation
Xue, X.; Asenbauer, J.; Eisenmann, T.; Lepore, G. O.; d’Acapito, F.; Xing, S.; Tübke, J.; Mullaliu, A.; Li, Y.; Geiger, D.; Biskupek, J.; Kaiser, U.; Steinle, D.; Birrozzi, A.; Bresser, D.
2024. Small Structures, 5 (6), Art.-Nr.: 2300545. doi:10.1002/sstr.202300545Static theoretical investigations of organic redox active materials for redox flow batteries
Zaichenko, A.; Achazi, A. J.; Kunz, S.; Wegner, H. A.; Janek, J.; Mollenhauer, D.
2024. Progress in Energy, 6, Article no: 012001. doi:10.1088/2516-1083/ad0913Unravelling the peculiar role of Co and Al in highly Ni-rich layered oxide cathode materials
Zhang, J.; Wang, S.; Yang, X.; Liu, Y.; Wu, Z.; Li, H.; Indris, S.; Ehrenberg, H.; Hua, W.
2024. Chemical Engineering Journal, 484, Article no: 149599. doi:10.1016/j.cej.2024.149599Modeling storage particle delamination and electrolyte cracking in cathodes of solid state batteries
Zhang, T.; Kamlah, M.; McMeeking, R. M.
2024. Journal of the Mechanics and Physics of Solids, 185, 105551. doi:10.1016/j.jmps.2024.105551 -
2023Reversible Electrodeposition of Potassium‐bridged Molecular Vanadium Oxides: A New Approach Towards Multi‐Electron Storage
Arya, N.; Philipp, T.; Greiner, S.; Steiner, M.; Kranz, C.; Anjass, M.
2023. Angewandte Chemie International Edition, 62 (35). doi:10.1002/anie.202306170Batteriesysteme der Zukunft - Foresight & Technikfolgenabschätzung: Monitoring November 2023
Baumann, M.; Weil, M.
2023. Verlag des Ita Wegman Instituts (ITA)Rhombohedral (R ) Prussian White as Cathode Material: An Ab‐initio Study
Baumgart, S.; Sotoudeh, M.; Groß, A.
2023. Batteries & Supercaps, 6 (12), e202300294. doi:10.1002/batt.202300294Societal acceptability of large stationary battery storage systems
Baur, D.; Baumann, M. J.; Stuhm, P.; Weil, M.
2023. Energy Technology, 11 (6), Art.-Nr.: 2201454. doi:10.1002/ente.202201454Multi‐Component PtFeCoNi Core‐Shell Nanoparticles on MWCNTs as Promising Bifunctional Catalyst for Oxygen Reduction and Oxygen Evolution Reactions
Braun, T.; Dinda, S.; Karkera, G.; Melinte, G.; Diemant, T.; Kübel, C.; Fichtner, M.; Pammer, F.
2023. ChemistrySelect, 8 (29), Art.-Nr.: e202300396. doi:10.1002/slct.202300396The role of exact exchange on the structure of water dimer radical cation: Hydrogen bond vs hemibond
Busch, M.; Sotoudeh, M.
2023. The Journal of Chemical Physics, 159 (3), Article no: 034303. doi:10.1063/5.0153759Characterization of the solid/electrolyte interphase at hard carbon anodes via scanning (electrochemical) probe microscopy
Daboss, S.; Philipp, T.; Palanisamy, K.; Flowers, J.; Stein, H. S.; Kranz, C.
2023. Electrochimica Acta, 453, Art.-Nr.: 142345. doi:10.1016/j.electacta.2023.142345Exploring the influence of FIB processing and SEM imaging on solid-state electrolytes
Ding, Z.; Tang, Y.; Chakravadhanula, V. S. K.; Ma, Q.; Tietz, F.; Dai, Y.; Scherer, T.; Kübel, C.
2023. Microscopy, 72 (4), 326–335. doi:10.1093/jmicro/dfac064The Impact of Microstructure on Filament Growth at the Sodium Metal Anode in All‐Solid‐State Sodium Batteries
Ding, Z.; Tang, Y.; Ortmann, T.; Eckhardt, J. K.; Dai, Y.; Rohnke, M.; Melinte, G.; Heiliger, C.; Janek, J.; Kübel, C.
2023. Advanced Energy Materials, 13 (48), Art.Nr.: 2302322. doi:10.1002/aenm.202302322Closing gaps in LCA of lithium-ion batteries: LCA of lab-scale cell production with new primary data
Erakca, M.; Pinto Bautista, S.; Moghaddas, S.; Baumann, M.; Bauer, W.; Leuthner, L.; Weil, M.
2023. Journal of Cleaner Production, 384, Art.-Nr.: 135510. doi:10.1016/j.jclepro.2022.135510Reactive Metals as Energy Storage and Carrier Media
Ersoy, H.; Baumann, M.; Weil, M.; Barelli, L.; Passerini, S.
2023. Sustainable Energy Storage in the Scope of Circular Economy – Advanced Materials and Device Design. Ed.: C. Costa, 17–41, John Wiley and Sons. doi:10.1002/9781119817741.ch2Development of a Mg/O ReaxFF Potential to describe the Passivation Processes in Magnesium‐Ion Batteries
Fiesinger, F.; Gaissmaier, D.; van den Borg, M.; Beßner, J.; van Duin, A. C. T.; Jacob, T.
2023. ChemSusChem, 16 (3), Art.-Nr.: e202201821. doi:10.1002/cssc.202201821Guest Ion-Dependent Reaction Mechanisms of New Pseudocapacitive Mg V (PO ) /Carbon Composite as Negative Electrode for Monovalent-Ion Batteries
Fu, Q.; Schwarz, B.; Ding, Z.; Sarapulova, A.; Weidler, P. G.; Missyul, A.; Etter, M.; Welter, E.; Hua, W.; Knapp, M.; Dsoke, S.; Ehrenberg, H.
2023. Advanced Science, 10 (11), Art.-Nr.: 2207283. doi:10.1002/advs.202207283To be or not to be – Is MgSc2Se4 a Mg-Ion Solid Electrolyte?
Glaser, C.; Wei, Z.; Indris, S.; Klement, P.; Chatterjee, S.; Ehrenberg, H.; Zhao-Karger, Z.; Rohnke, M.; Janek, J.
2023. Advanced Energy Materials, Art.-Nr.: 2301980. doi:10.1002/aenm.202301980Challenges for ab initio molecular dynamics simulations of electrochemical interfaces
Groß, A.
2023. Current Opinion in Electrochemistry, 40, Article no: 101345. doi:10.1016/j.coelec.2023.101345Experimental and Computational Aspects of Electrochemical Reflection Anisotropy Spectroscopy : A Review
Guidat, M.; Löw, M.; Kölbach, M.; Kim, J.; May, M. M.
2023. ChemElectroChem, 10 (8), e2023000. doi:10.1002/celc.202300027Deciphering Electrolyte Degradation in Sodium-Based Batteries: The Role of Conductive Salt Source, Additives, and Storage Condition
Hashimov, M.; Hofmann, A.
2023. Batteries, 9 (11), Art.-Nr. 530. doi:10.3390/batteries9110530Asymptotic properties of one-layer artificial neural networks with sparse connectivity
Hirsch, C.; Neumann, M.; Schmidt, V.
2023. Statistics & Probability Letters, 193, Art.-Nr.: 109698. doi:10.1016/j.spl.2022.109698Revealing the Formation of Dialkyl Dioxahexane Dioate Products from Ethylene Carbonate Based Electrolytes on Lithium and Potassium Surfaces
Hofmann, A.; Müller, F.; Schöner, S.; Jeschull, F.
2023. Batteries & Supercaps, 6 (12), Art.Nr.: e202300325. doi:10.1002/batt.202300325Dynamic inconsistency between electrochemical reaction and phase transition in Na-deficient layered cathode materials
Hua, W.; Yang, X.; Wang, S.; Li, H.; Senyshyn, A.; Tayal, A.; Baran, V.; Chen, Z.; Avdeev, M.; Knapp, M.; Ehrenberg, H.; Saadoune, I.; Chou, S.; Indris, S.; Guo, X.
2023. Energy Storage Materials, 61, Article no: 102906. doi:10.1016/j.ensm.2023.102906Multivalent Cation Transport in Polymer Electrolytes – Reflections on an Old Problem
Jeschull, F.; Hub, C.; Kolesnikov, T. I.; Sundermann, D.; Hernández, G.; Voll, D.; Mindemark, J.; Théato, P.
2023. Advanced Energy Materials. doi:10.1002/aenm.202302745P3 type layered oxide frameworks: An appealing family of insertion materials for K-ion batteries
Jha, P. K.; Pralong, V.; Fichtner, M.; Barpanda, P.
2023. Current Opinion in Electrochemistry, 38, Art.-Nr.: 101216. doi:10.1016/j.coelec.2023.101216Entropic Contributions to Sodium Solvation and Solvent Stabilization upon Electrochemical Sodium deposition from Diglyme and Propylene Carbonate Electrolytes
Karcher, F.; Uhl, M.; Geng, T.; Jacob, T.; Schuster, R.
2023. Angewandte Chemie International Edition, 62 (22), e202301253. doi:10.1002/anie.202301253From lithium to potassium: Comparison of cations in poly(ethylene oxide)-based block copolymer electrolytes for solid-state alkali metal batteries
Khudyshkina, A. D.; Butzelaar, A. J.; Guo, Y.; Hoffmann, M.; Bergfeldt, T.; Schaller, M.; Indris, S.; Wilhelm, M.; Théato, P.; Jeschull, F.
2023. Electrochimica Acta, 454, Article no: 142421. doi:10.1016/j.electacta.2023.142421Atomic Layer Deposition Derived Zirconia Coatings on Ni‐Rich Cathodes in Solid‐State Batteries: Correlation Between Surface Constitution and Cycling Performance
Kitsche, D.; Tang, Y.; Hemmelmann, H.; Walther, F.; Bianchini, M.; Kondrakov, A.; Janek, J.; Brezesinski, T.
2023. Small Science, 3 (2), Art.-Nr.: 2200073. doi:10.1002/smsc.202200073Drying of Compact and Porous NCM Cathode Electrodes in Different Multilayer Architectures: Influence of Layer Configuration and Drying Rate on Electrode Properties
Klemens, J.; Burger, D.; Schneider, L.; Spiegel, S.; Müller, M.; Bohn, N.; Bauer, W.; Ehrenberg, H.; Scharfer, P.; Schabel, W.
2023. Energy Technology, 11 (8), Art.Nr.: 2300267. doi:10.1002/ente.202300267Process and Drying Behavior Toward Higher Drying Rates of Hard Carbon Anodes for Sodium‐Ion Batteries with Different Particle Sizes: An Experimental Study in Comparison to Graphite for Lithium‐Ion‐Batteries
Klemens, J.; Schneider, L.; Burger, D.; Zimmerer, N.; Müller, M.; Bauer, W.; Ehrenberg, H.; Scharfer, P.; Schabel, W.
2023. Energy Technology, 11 (8), Art.-Nr.: 2300338. doi:10.1002/ente.202300338Challenges and Opportunities for Large‐Scale Electrode Processing for Sodium‐Ion and Lithium‐Ion Battery
Klemens, J.; Wurba, A.-K.; Burger, D.; Müller, M.; Bauer, W.; Büchele, S.; Leonet, O.; Blázquez, J. A.; Boyano, I.; Ayerbe, E.; Ehrenberg, H.; Fleischer, J.; Smith, A.; Scharfer, P.; Schabel, W.
2023. Batteries & Supercaps, 6 (11), Art.Nr.: e202300291. doi:10.1002/batt.202300291Investigation of SnS₂‐rGO Sandwich Structures as Negative Electrode for Sodium‐ion and Potassium‐ion Batteries
Li, C.; Pfeifer, K.; Luo, X.; Melinte, G.; Wang, J.; Zhang, Z.; Zhang, Y.; Dong, P.; Sarapulova, A.; Ehrenberg, H.; Dsoke, S.
2023. ChemSusChem, 16 (7), e202202281. doi:10.1002/cssc.202202281Chemistry, electrochemistry, and electrochemical applications of magnesium
Li, Z.; Wang, L.; Bautista, S. P.; Weil, M.
2023. Reference Module in Chemistry, Molecular Sciences and Chemical Engineering. Ed.: J. Reedijk, Elsevier. doi:10.1016/B978-0-323-96022-9.00066-9ToF-SIMS in battery research: Advantages, limitations, and best practices
Lombardo, T.; Walther, F.; Kern, C.; Moryson, Y.; Weintraut, T.; Henss, A.; Rohnke, M.
2023. Journal of Vacuum Science & Technology A, 41 (5), Article no: 053207. doi:10.1116/6.0002850Fundamental Understanding and Quantification of Capacity Losses Involving the Negative Electrode in Sodium‐Ion Batteries
Ma, L. A.; Buckel, A.; Hofmann, A.; Nyholm, L.; Younesi, R.
2023. Advanced Science, Art.-Nr.2306771. doi:10.1002/advs.202306771Capacity losses due to solid electrolyte interphase formation and sodium diffusion in sodium-ion batteries
Ma, L. A.; Buckel, A.; Nyholm, L.; Younesi, R.
2023. Research Square. doi:10.21203/rs.3.rs-623903/v1Environmental life cycle assessment of emerging solid-state batteries: A review
Mandade, P.; Weil, M.; Baumann, M.; Wei, Z.
2023. Chemical engineering journal advances, 13, Art.-Nr.: 100439. doi:10.1016/j.ceja.2022.100439Sodium Insertion into Fe[Fe(CN) 6 ] Framework Prepared by Microwave‐Assisted Co‐Precipitation
Maroni, F.; Li, M.; Dongmo, S.; Gauckler, C.; Wohlfahrt-Mehrens, M.; Giorgetti, M.; Marinaro, M.
2023. ChemElectroChem, 10 (8), e202201070. doi:10.1002/celc.202201070New insights into Self‐discharge and Heat Generation in Magnesium Batteries
Mohsin, I. U.; Riedel, S.; Xiu, Y.; Zhao-Karger, Z.; Ziebert, C.
2023. Batteries & Supercaps, 6 (7), Art.Nr.: e202300137. doi:10.1002/batt.202300137Enabling the Electrochemical Performance of Maricite-NaMnPO4 and Maricite-NaFePO4 Cathode Materials in Sodium-Ion Batteries
Mohsin, I. U.; Schneider, L.; Yu, Z.; Cai, W.; Ziebert, C.
2023. (A. Arunachalam, Hrsg.) International Journal of Electrochemistry, 2023, Artlk. ID.: 6054452. doi:10.1155/2023/6054452Effect of self and extrinsic encapsulation on electron resilience of porous 2D polymer nanosheets
Mücke, D.; Linck, M.; Guzzinati, G.; Müller, H.; Levin, B. D. A.; Bammes, B. E.; Brouwer, R. G.; Jelezko, F.; Qi, H.; Kaiser, U.
2023. Micron, 174, Article no: 103525. doi:10.1016/j.micron.2023.103525Influences on Reliable Capacity Measurements of Hard Carbon in Highly Loaded Electrodes
Müller, C.; Wang, Z.; Hofmann, A.; Stueble, P.; Liu-Théato, X.; Klemens, J.; Smith, A.
2023. Batteries & Supercaps, 6 (11), Art.Nr.: e202300322. doi:10.1002/batt.202300322Morphology‐Dependent Influences on the Performance of Battery Cells with a Hierarchically Structured Positive Electrode**
Naumann, J.; Bohn, N.; Birkholz, O.; Neumann, M.; Müller, M.; Binder, J. R.; Kamlah, M.
2023. Batteries & Supercaps, 6 (12), Art.-Nr.: e202300264. doi:10.1002/batt.202300264A data-driven modeling approach to quantify morphology effects on transport properties in nanostructured NMC particles
Neumann, M.; Wetterauer, S. E.; Osenberg, M.; Hilger, A.; Gräfensteiner, P.; Wagner, A.; Bohn, N.; Binder, J. R.; Manke, I.; Carraro, T.; Schmidt, V.
2023. International Journal of Solids and Structures, 280, Article no: 112394. doi:10.1016/j.ijsolstr.2023.112394Open Challenges on Aluminum Triflate-Based Electrolytes for Aluminum Batteries
Rahide, F.; Zemlyanushin, E.; Bosch, G.-M.; Dsoke, S.
2023. Journal of The Electrochemical Society, 170 (3), Article no: 030546. doi:10.1149/1945-7111/acc762Conductivity experiments for electrolyte formulations and their automated analysis
Rahmanian, F.; Vogler, M.; Wölke, C.; Yan, P.; Fuchs, S.; Winter, M.; Cekic-Laskovic, I.; Stein, H. S.
2023. Scientific Data, 10 (1), Art.-Nr.: 43. doi:10.1038/s41597-023-01936-3Surface Properties‐Performance Relationship of Aluminum Foil as Negative Electrode for Rechargeable Aluminum Batteries
Sabi, N.; Palanisamy, K.; Rahide, F.; Daboss, S.; Kranz, C.; Dsoke, S.
2023. Batteries & Supercaps, 6 (11), Art.Nr.: e202300298. doi:10.1002/batt.202300298Unraveling Propylene Oxide Formation in Alkali Metal Batteries
Stottmeister, D.; Wildersinn, L.; Maibach, J.; Hofmann, A.; Jeschull, F.; Groß, A.
2023. ChemSusChem, 17 (3), Art.Nr.: e202300995. doi:10.1002/cssc.202300995On a high-capacity aluminium battery with a two-electron phenothiazine redox polymer as positive electrode
Studer, G.; Schmidt, A.; Büttner, J.; Schmidt, M.; Fischer, A.; Krossing, I.; Esser, B.
2023. Energy & Environmental Science. doi:10.1039/D3EE00235GNew Insights into Self‐Discharge and Heat Generation in Magnesium Batteries
Ul Mohsin, I.; Riedel, S.; Xiu, Y.; Zhao-Karger, Z.; Ziebert, C.
2023. Batteries & Supercaps, 6 (7), Art.-Nr.: e202300251. doi:10.1002/batt.202300251Brokering between tenants for an international materials acceleration platform
Vogler, M.; Busk, J.; Hajiyani, H.; Jørgensen, P. B.; Safaei, N.; Castelli, I. E.; Ramirez, F. F.; Carlsson, J.; Pizzi, G.; Clark, S.; Hanke, F.; Bhowmik, A.; Stein, H. S.
2023. Matter, 6 (9), 2647–2665. doi:10.1016/j.matt.2023.07.016Synergy of cations in high entropy oxide lithium ion battery anode
Wang, K.; Hua, W.; Huang, X.; Stenzel, D.; Wang, J.; Ding, Z.; Cui, Y.; Wang, Q.; Ehrenberg, H.; Breitung, B.; Kübel, C.; Mu, X.
2023. Nature Communications, 14, Art.-Nr.: 1487. doi:10.1038/s41467-023-37034-6Segmentation and morphological analysis of amyloid fibrils from cryo-EM image data
Weber, M.; Neumann, M.; Schmidt, M.; Pfeiffer, P. B.; Bansal, A.; Fändrich, M.; Schmidt, V.
2023. Journal of Mathematics in Industry, 13 (2). doi:10.1186/s13362-023-00131-8In Situ Observation of Room‐Temperature Magnesium Metal Deposition on a NASICON/IL Hybrid Solid Electrolyte
Wei, Z.; Singh, D. K.; Helmbrecht, K.; Sann, J.; Yusim, Y.; Kieser, J. A.; Glaser, C.; Rohnke, M.; Groß, A.; Janek, J.
2023. Advanced Energy Materials, 13 (44), Art.-Nr.: 2302525. doi:10.1002/aenm.202302525Anion Storage Chemistry of Organic Cathodes for High‐Energy and High‐Power Density Divalent Metal Batteries
Xiu, Y.; Mauri, A.; Dinda, S.; Pramudya, Y.; Ding, Z.; Diemant, T.; Sarkar, A.; Wang, L.; Li, Z.; Wenzel, W.; Fichtner, M.; Zhao-Karger, Z.
2023. Angewandte Chemie International Edition, 62 (2), Art.: e202212339. doi:10.1002/anie.202212339Conformal Li HfO /HfO Nanoparticle Coatings on Layered Ni-Rich Oxide Cathodes for Stabilizing Interfaces in All-Solid-State Batteries
Zhang, R.; Ma, Y.; Tang, Y.; Goonetilleke, D.; Diemant, T.; Janek, J.; Kondrakov, A.; Brezesinski, T.
2023. Chemistry of Materials, 35 (17), 6835–6844. doi:10.1021/acs.chemmater.3c01116Identification of Lithium Compounds on Surfaces of Lithium Metal Anode with Machine-Learning-Assisted Analysis of ToF-SIMS Spectra
Zhao, Y.; Otto, S.-K.; Lombardo, T.; Henss, A.; Koeppe, A.; Selzer, M.; Janek, J.; Nestler, B.
2023. ACS Applied Materials & Interfaces, 15 (43), 50469 – 50478. doi:10.1021/acsami.3c09643 -
2022Comparing the Solid Electrolyte Interphases on Graphite Electrodes in K and Li Half Cells
Allgayer, F.; Maibach, J.; Jeschull, F.
2022. ACS applied energy materials, 5 (1), 1136–1148. doi:10.1021/acsaem.1c03491A Roadmap for Transforming Research to Invent the Batteries of the Future Designed within the European Large Scale Research Initiative BATTERY 2030+
Amici, J.; Asinari, P.; Ayerbe, E.; Barboux, P.; Bayle-Guillemaud, P.; Behm, R. J.; Berecibar, M.; Berg, E.; Bhowmik, A.; Bodoardo, S.; Castelli, I. E.; Cekic-Laskovic, I.; Christensen, R.; Clark, S.; Diehm, R.; Dominko, R.; Fichtner, M.; Franco, A. A.; Grimaud, A.; Guillet, N.; Hahlin, M.; Hartmann, S.; Heiries, V.; Hermansson, K.; Heuer, A.; Jana, S.; Jabbour, L.; Kallo, J.; Latz, A.; Lorrmann, H.; Løvvik, O. M.; Lyonnard, S.; Meeus, M.; Paillard, E.; Perraud, S.; Placke, T.; Punckt, C.; Raccurt, O.; Ruhland, J.; Sheridan, E.; Stein, H.; Tarascon, J.-M.; Trapp, V.; Vegge, T.; Weil, M.; Wenzel, W.; Winter, M.; Wolf, A.; Edström, K.
2022. Advanced Energy Materials, 12 (17), Art.-Nr.: 2102785. doi:10.1002/aenm.202102785Comprehensive Approach to Investigate the De‐/Lithiation Mechanism of Fe‐Doped SnO₂ as Lithium‐Ion Anode Material
Asenbauer, J.; Wirsching, A.-L.; Lang, M.; Indris, S.; Eisenmann, T.; Mullaliu, A.; Birrozzi, A.; Hoefling, A.; Geiger, D.; Kaiser, U.; Schuster, R.; Bresser, D.
2022. Advanced Sustainable Systems, 6 (8), Artkl. Nr.: 2200102. doi:10.1002/adsu.202200102Charging sustainable batteries
Bauer, C.; Burkhardt, S.; Dasgupta, N. P.; Ellingsen, L. A.-W.; Gaines, L. L.; Hao, H.; Hischier, R.; Hu, L.; Huang, Y.; Janek, J.; Liang, C.; Li, H.; Li, J.; Li, Y.; Lu, Y.-C.; Luo, W.; Nazar, L. F.; Olivetti, E. A.; Peters, J. F.; Rupp, J. L. M.; Weil, M.; Whitacre, J. F.; Xu, S.
2022. Nature Sustainability, 5 (3), 176–178. doi:10.1038/s41893-022-00864-1High-Throughput Experimentation and Computational Freeway Lanes for Accelerated Battery Electrolyte and Interface Development Research
Benayad, A.; Diddens, D.; Heuer, A.; Krishnamoorthy, A. N.; Maiti, M.; Cras, F. L.; Legallais, M.; Rahmanian, F.; Shin, Y.; Stein, H.; Winter, M.; Wölke, C.; Yan, P.; Cekic-Laskovic, I.
2022. Advanced Energy Materials, 12 (17), Art.Nr.: 2102678. doi:10.1002/aenm.202102678Managing FAIR Tribological Data Using Kadi4Mat
Brandt, N.; Garabedian, N. T.; Schoof, E.; Schreiber, P. J.; Zschumme, P.; Greiner, C.; Selzer, M.
2022. Data, 7 (2), Art.-Nr. 15. doi:10.3390/data7020015Interaction of Mg with the ionic liquid 1-butyl-1-methylpyrrolidinium bis(trifluoromethylsulfonyl)imide—An experimental and computational model study of the electrode–electrolyte interface in post-lithium batteries
Buchner, F.; Forster-Tonigold, K.; Bolter, T.; Rampf, A.; Klein, J.; Groß, A.; Behm, R. J.
2022. Journal of vacuum science & technology / A, 40 (2), Artikel-Nr.: 023204. doi:10.1116/6.0001658Autonomous visual detection of defects from battery electrode manufacturing
Choudhary, N.; Clever, H.; Ludwigs, R.; Rath, M.; Gannouni, A.; Schmetz, A.; Hülsmann, T.; Sawodny, J.; Fischer, L.; Kampker, A.; Fleischer, J.; Stein, H. S.
2022. American Chemical Society (ACS). doi:10.26434/chemrxiv-2022-pvwj3From high‐pressure β‐V 2 O 5 to κ‐Na x V 2 O 5 (x = 0.4 – 0.55): a structural, chemical and kinetic insight into a sodiated phase with a large interlayer space
Córdoba, R.; Goclon, J.; Sarapulova, A.; Fu, Q.; Maibach, J.; Dsoke, S.; Fauth, F.; Kuhn, A.; García-Alvarado, F.
2022. Applied Research, 2 (1), Art.Nr. e202200052. doi:10.1002/appl.202200052Unravelling Charge Carrier Mobility in d₀ ‐Metal‐based Spinels
Dillenz, M.; Sotoudeh, M.; Glaser, C.; Janek, J.; Groß, A.; Euchner, H.
2022. Batteries & Supercaps, 5 (7), Art.-Nr. e202200164. doi:10.1002/batt.202200164Atomistic modeling of Li- and post-Li-ion batteries
Euchner, H.; Groß, A.
2022. Physical Review Materials, 6 (4), Article no: 040302. doi:10.1103/PhysRevMaterials.6.040302Rechargeable Batteries of the Future—The State of the Art from a BATTERY 2030+ Perspective
Fichtner, M.; Edström, K.; Ayerbe, E.; Berecibar, M.; Bhowmik, A.; Castelli, I. E.; Clark, S.; Dominko, R.; Erakca, M.; Franco, A. A.; Grimaud, A.; Horstmann, B.; Latz, A.; Lorrmann, H.; Meeus, M.; Narayan, R.; Pammer, F.; Ruhland, J.; Stein, H.; Vegge, T.; Weil, M.
2022. Advanced Energy Materials, 12 (17), 2102904. doi:10.1002/aenm.202102904Recent Research and Progress in Batteries for Electric Vehicles
Fichtner, M.
2022. Batteries and Supercaps, 5 (2), e202100224. doi:10.1002/batt.202100224First‐Principles Studies on the Atomistic Properties of Metallic Magnesium as Anode Material in Magnesium‐Ion Batteries
Fiesinger, F.; Gaissmaier, D.; Borg, M.; Jacob, T.
2022. ChemSusChem, 15 (14), Art.Nr. e202200414. doi:10.1002/cssc.202200414A Combined XPS and Computational Study of the Chemical Reduction of BMP‐TFSI by Lithium
Forster-Tonigold, K.; Buchner, F.; Bansmann, J.; Behm, R. J.; Groß, A.
2022. Batteries & Supercaps, 5 (12), Art._Nr: e202200484. doi:10.1002/batt.202200484A Combined XPS and Computational Study of the Chemical Reduction of BMP‐TFSI by Lithium
Forster-Tonigold, K.; Buchner, F.; Bansmann, J.; Behm, R. J.; Groß, A.
2022. Batteries and Supercaps, 5 (12), Art.Nr. e202200307. doi:10.1002/batt.202200307Preferred Site Occupation of Doping Cation and Its Impact on the Local Structure of V₂O₅
Fu, Q.; Hansen, A.-L.; Schwarz, B.; Sarapulova, A.; Zhu, L.; Tian, G.; Etter, M.; Missyul, A.; Welter, E.; Murzin, V.; Indris, S.; Azmi, R.; Knapp, M.; Dsoke, S.; Ehrenberg, H.
2022. Chemistry of Materials, 34 (22), 9844–9853. doi:10.1021/acs.chemmater.2c01695High‐Voltage Aqueous Mg‐Ion Batteries Enabled by Solvation Structure Reorganization
Fu, Q.; Wu, X.; Luo, X.; Indris, S.; Sarapulova, A.; Bauer, M.; Wang, Z.; Knapp, M.; Ehrenberg, H.; Wei, Y.; Dsoke, S.
2022. Advanced functional materials, 32 (16), Art.Nr.: 2110674. doi:10.1002/adfm.202110674V₂O₅ as a versatile electrode material for postlithium energy storage systems
Fu, Q.; Zhao, H.; Sarapulova, A.; Dsoke, S.
2022. Applied Research, 2 (3), Art.Nr.: e202200070. doi:10.1002/appl.202200070Detailed Structural and Electrochemical Comparison between High Potential Layered P2-NaMnNi and Doped P2-NaMnNiMg Oxides
Gauckler, C.; Dillenz, M.; Maroni, F.; Pfeiffer, L. F.; Biskupek, J.; Sotoudeh, M.; Fu, Q.; Kaiser, U.; Dsoke, S.; Euchner, H.; Axmann, P.; Wohlfahrt-Mehrens, M.; Groß, A.; Marinaro, M.
2022. ACS Applied Energy Materials, 5 (11), 13735–13750. doi:10.1021/acsaem.2c02402Reversible vs Standard Hydrogen Electrode Scale in Interfacial Electrochemistry from a Theoretician’s Atomistic Point of View
Groß, A.
2022. The Journal of Physical Chemistry C, 126 (28), 11439–11446. doi:10.1021/acs.jpcc.2c02734Ab Initio Simulations of Water/Metal Interfaces
Groß, A.; Sakong, S.
2022. Chemical Reviews, 122 (12), 10746–10776. doi:10.1021/acs.chemrev.1c00679Revisiting the Chevrel Phase: Impact of Dispersion Corrections on the Properties of Mo6S8 for Cathode Applications**
Helmbrecht, K.; Euchner, H.; Groß, A.
2022. Batteries & Supercaps, 5 (8), Art.Nr. e202200002. doi:10.1002/batt.202200002Initial Stages of Sodium Deposition onto Au(111) from [MPPip][TFSI]: An In‐Situ STM Study for Sodium‐Ion Battery Electrolytes
Heubach, M.-K.; Schuett, F. M.; Kibler, L. A.; Abdelrahman, A.; Jacob, T.
2022. ChemElectroChem, 9 (20), Art.Nr.: e202200722. doi:10.1002/celc.202200722Novel Phosphonium-Based Ionic Liquid Electrolytes for Battery Applications
Hofmann, A.; Rauber, D.; Wang, T.-M.; Hempelmann, R.; Kay, C. W. M.; Hanemann, T.
2022. Molecules, 27 (15), Art.Nr.: 4729. doi:10.3390/molecules27154729Dataset of propylene carbonate based liquid electrolyte mixtures for sodium-ion cells
Hofmann, A.; Wang, Z.; Bautista, S. P.; Weil, M.; Müller, F.; Löwe, R.; Schneider, L.; Mohsin, I. U.; Hanemann, T.
2022. Data in Brief, 40, Article no: 107775. doi:10.1016/j.dib.2021.107775Advancing the Sustainability of Batteries. A Tongji University/Nature Sustainability Expert Panel Report
Huang, Y.; Bauer, C.; Burkhardt, S.; Dasgupta, N. P.; Ellingsen, L. A.-W.; Gaines, L. L.; Hao, H.; Hischier, R.; Hu, L.; Huang, Y.-M.; Janek, J.; Liang, C.; Li, H.; Li, J.; Li, Y.; Lu, Y.-C.; Luo, W.; Nazar, L. F.; Olivetti, E. A.; Peters, J. F.; Rupp, J. L. M.; Weil, M.; Whitacre, J. F.; Xu, S.
2022. Tongji UniversityDevelopment of Magnesium Borate Electrolytes: Explaining the Success of Mg[B(hfip)4]2 Salt
Jankowski, P.; Li, Z.; Zhao-Karger, Z.; Diemant, T.; Fichtner, M.; Vegge, T.; Lastra, J. M. G.
2022. Energy storage materials, 45, 1133–1143. doi:10.1016/j.ensm.2021.11.012Poly(ethylene oxide)-Based Electrolytes for Solid-State Potassium Metal Batteries with a Prussian Blue Positive Electrode
Khudyshkina, A. D.; Morozova, P. A.; Butzelaar, A. J.; Hoffmann, M.; Wilhelm, M.; Theato, P.; Fedotov, S. S.; Jeschull, F.
2022. ACS Applied Polymer Materials, 4 (4), 2734–2746. doi:10.1021/acsapm.2c00014Drying of NCM Cathode Electrodes with Porous, Nanostructured Particles Versus Compact Solid Particles: Comparative Study of Binder Migration as a Function of Drying Conditions
Klemens, J.; Schneider, L.; Herbst, E. C.; Bohn, N.; Müller, M.; Bauer, W.; Scharfer, P.; Schabel, W.
2022. Energy technology, 10 (4), Article no: 2100985. doi:10.1002/ente.202100985Simulation-Based and Data-Driven Techniques for Quantifying the Influence of the Carbon Binder Domain on Electrochemical Properties of Li-Ion Batteries
Knorr, T.; Hein, S.; Prifling, B.; Neumann, M.; Danner, T.; Schmidt, V.; Latz, A.
2022. Energies, 15 (21), Art.-Nr.: 7821. doi:10.3390/en15217821The interfacial structure of InP(100) in contact with HCl and H2SO4 studied by reflection anisotropy spectroscopy
Löw, M.; Guidat, M.; Kim, J.; May, M. M.
2022. RSC Advances, 12 (50), 32756–32764. doi:10.1039/D2RA05159AResolving the Role of Configurational Entropy in Improving Cycling Performance of Multicomponent Hexacyanoferrate Cathodes for Sodium‐Ion Batteries
Ma, Y.; Hu, Y.; Pramudya, Y.; Diemant, T.; Wang, Q.; Goonetilleke, D.; Tang, Y.; Zhou, B.; Hahn, H.; Wenzel, W.; Fichtner, M.; Ma, Y.; Breitung, B.; Brezesinski, T.
2022. Advanced Functional Materials, 32 (34), Art.Nr. 2202372. doi:10.1002/adfm.202202372Advances in Nanomaterials for Lithium-Ion/Post-Lithium-Ion Batteries and Supercapacitors
Marinaro, M.; Dsoke, S.
2022. Nanomaterials, 12 (15), Art.Nr. 2512. doi:10.3390/nano12152512Sodiation of hard carbon: how separating enthalpy and entropy contributions can find transitions hidden in the voltage profile
Mercer, M.; Affleck, S.; Gavilan-Arriazu, E. M.; Zulke, A. A.; Maughan, P. A.; Trivedi, S.; Fichtner, M.; Reddy Munnangi, A.; Leiva, E. P. M.; Hoster, H. E.
2022. ChemPhysChem, 23 (5), e202100748. doi:10.1002/cphc.202100748Heat generation and degradation mechanisms studied on Na₃V₂(PO₄)₃/C positive electrode material in full pouch / coin cell assembly
Mohsin, I. U.; Schneider, L.; Häringer, M.; Ziebert, C.; Rohde, M.; Bauer, W.; Ehrenberg, H.; Seifert, H. J.
2022. Journal of Power Sources, 545, Art.-Nr.: 231901. doi:10.1016/j.jpowsour.2022.2319013D microstructure characterization of polymer battery electrodes by statistical image analysis based on synchrotron X-ray tomography
Neumann, M.; Ademmer, M.; Osenberg, M.; Hilger, A.; Wilde, F.; Muench, S.; Hager, M. D.; Schubert, U. S.; Manke, I.; Schmidt, V.
2022. Journal of Power Sources, 542, 231783. doi:10.1016/j.jpowsour.2022.231783Classification of FIB/SEM-tomography images for highly porous multiphase materials using random forest classifiers
Osenberg, M.; Hilger, A.; Neumann, M.; Wagner, A.; Bohn, N.; Binder, J. R.; Schmidt, V.; Banhart, J.; Manke, I.
2022. arxiv. doi:10.48550/arXiv.2207.14114Layered P2-Na Mn Ni O Cathode Materials For Sodium-Ion Batteries: Synthesis, Electrochemistry and Influence of Ambient Storage
Pfeiffer, L. F.; Jobst, N.; Gauckler, C.; Lindén, M.; Marinaro, M.; Passerini, S.; Wohlfahrt-Mehrens, M.; Axmann, P.
2022. Frontiers in Energy Research, 10, Art.-Nr.: 910842. doi:10.3389/fenrg.2022.910842Visualization of structural changes and degradation of porphyrin-based battery electrodes
Philipp, T.; Neusser, G.; Abouzari-Lotf, E.; Shakouri, S.; Wilke, F. D. H.; Fichtner, M.; Ruben, M.; Mundszinger, M.; Biskupek, J.; Kaiser, U.; Scheitenberger, P.; Lindén, M.; Kranz, C.
2022. Journal of Power Sources, 522, Art.-Nr.: 231002. doi:10.1016/j.jpowsour.2022.231002Quantitative Comparison of Different Approaches for Reconstructing the Carbon‐Binder Domain from Tomographic Image Data of Cathodes in Lithium‐Ion Batteries and Its Influence on Electrochemical Properties
Prifling, B.; Neumann, M.; Hein, S.; Danner, T.; Heider, E.; Hoffmann, A.; Rieder, P.; Hilger, A.; Osenberg, M.; Manke, I.; Wohlfahrt-Mehrens, M.; Latz, A.; Schmidt, V.
2022. Energy Technology, Art.-Nr.: 2200784. doi:10.1002/ente.202200784Enabling Modular Autonomous Feedback-Loops in Materials Science through Hierarchical Experimental Laboratory Automation and Orchestration
Rahmanian, F.; Flowers, J.; Guevarra, D.; Richter, M.; Fichtner, M.; Donnely, P.; Gregoire, J. M.; Stein, H. S.
2022. Advanced Materials Interfaces, 8 (9), 2101987. doi:10.1002/admi.202101987One-shot active learning for globally optimal battery electrolyte conductivity
Rahmanian, F.; Vogler, M.; Wölke, C.; Yan, P.; Winter, M.; Cekic-Laskovic, I.; Stein, H. S.
2022. American Chemical Society (ACS). doi:10.26434/chemrxiv-2022-1z8gnInvestigation of the Anode-Electrolyte Interface in a Magnesium Full-Cell with Fluorinated Alkoxyborate-Based Electrolyte
Roy, A.; Bhagavathi Parambath, V.; Diemant, T.; Neusser, G.; Kranz, C.; Behm, R. J.; Li, Z.; Zhao-Karger, Z.; Fichtner, M.
2022. Batteries and Supercaps, 5 (4), Art.-Nr.: e202100305. doi:10.1002/batt.202100305Transport Properties in Electrodes for Lithium-Ion Batteries: Comparison of Compact versus Porous NCM Particles
Schneider, L.; Klemens, J.; Herbst, E. C.; Müller, M.; Scharfer, P.; Schabel, W.; Bauer, W.; Ehrenberg, H.
2022. Journal of The Electrochemical Society, 169 (10), Art.-Nr.: 100553. doi:10.1149/1945-7111/ac9c37Suitability of Carbazolyl Hauser and Turbo‐Hauser Bases as Magnesium‐Based Electrolytes
Schüler, P.; Sengupta, S.; Zaubitzer, S.; Fiesinger, F.; Dongmo, S.; Görls, H.; Wohlfahrt-Mehrens, M.; Borg, M.; Gaissmaier, D.; Krieck, S.; Marinaro, M.; Jacob, T.; Westerhausen, M.
2022. European Journal of Inorganic Chemistry, 2022 (17), Art.-Nr.: e202200149. doi:10.1002/ejic.202200149Stability of magnesium binary and ternary compounds for batteries determined from first principles
Sotoudeh, M.; Gross, A.
2022. American Chemical Society (ACS). doi:10.26434/chemrxiv-2022-m90n5Descriptor and Scaling Relations for Ion Mobility in Crystalline Solids
Sotoudeh, M.; Groß, A.
2022. JACS Au, 2 (2), 463–471. doi:10.1021/jacsau.1c00505Advancing data-driven chemistry by beating benchmarks
Stein, H. S.
2022. Trends in Chemistry, 4 (8), 682–684. doi:10.1016/j.trechm.2022.05.003From materials discovery to system optimization by integrating combinatorial electrochemistry and data science
Stein, H. S.; Sanin, A.; Rahmanian, F.; Zhang, B.; Vogler, M.; Flowers, J. K.; Fischer, L.; Fuchs, S.; Choudhary, N.; Schroeder, L.
2022. Current Opinion in Electrochemistry, 35, Art.-Nr.: 101053. doi:10.1016/j.coelec.2022.101053Brokering between tenants for an international materials acceleration platform
Vogler, M.; Busk, J.; Hajiyani, H.; Jørgensen, P. B.; Safaei, N.; Castelli, I.; Ramírez, F. F.; Carlsson, J.; Pizzi, G.; Clark, S.; Hanke, F.; Bhowmik, A.; Stein, H. S.
2022. American Chemical Society (ACS). doi:10.26434/chemrxiv-2022-grgrdP2-type layered high-entropy oxides as sodium-ion cathode materials
Wang, J.; Dreyer, S. L.; Wang, K.; Ding, Z.; Diemant, T.; Karkera, G.; Ma, Y.; Sarkar, A.; Zhou, B.; Gorbunov, M. V.; Omar, A.; Mikhailova, D.; Presser, V.; Fichtner, M.; Hahn, H.; Brezesinski, T.; Breitung, B.; Wang, Q.
2022. Materials Futures, 1 (3), Art.Nr. 035104. doi:10.1088/2752-5724/ac8ab9A Novel and Highly Efficient Indolyl‐Based Electrolyte for Mg Batteries
Zaubitzer, S.; Dongmo, S.; Schüler, P.; Krieck, S.; Fiesinger, F.; Gaissmaier, D.; van den Borg, M.; Jacob, T.; Westerhausen, M.; Wohlfahrt-Mehrens, M.; Marinaro, M.
2022. Energy Technology, 10 (8), Art.-Nr.: 2200440. doi:10.1002/ente.202200440Robotic cell assembly to accelerate battery research
Zhang, B.; Fischer, L.; Sanin, A.; Stein, H. S.
2022. American Chemical Society (ACS). doi:10.26434/chemrxiv-2022-vt2n9Combined Thermal Runaway Investigation of Coin Cells with an Accelerating Rate Calorimeter and a Tian-Calvet Calorimeter
Zhao, W.; Rohde, M.; Mohsin, I. U.; Ziebert, C.; Du, Y.; Seifert, H. J.
2022. Batteries, 8 (2). doi:10.3390/batteries8020015Study on Na₂V₀ ₆₇Mn₀ ₃₃Ti(PO₄)₃ electrodes with ultralow voltage hysteresis for high performance sodium-ion batteries
Zhao, Z.; Darma, M. S. D.; Tian, G.; Luo, X.; Zhao, E.; Wang, B.-T.; Zhao, J.; Hua, W.; Zhao, X.; Wang, Y.; Ehrenberg, H.; Dsoke, S.
2022. Chemical Engineering Journal, 444, Article no: 136608. doi:10.1016/j.cej.2022.136608Calcium-tin alloys as anodes for rechargeable non-aqueous calcium-ion batteries at room temperature
Zhao-Karger, Z.; Xiu, Y.; Li, Z.; Reupert, A.; Smok, T.; Fichtner, M.
2022. Nature Communications, 13 (1), 3849. doi:10.1038/s41467-022-31261-z -
2021A Self-Conditioned Metalloporphyrin as a Highly Stable Cathode for Fast Rechargeable Magnesium Batteries
Abouzari-Lotf, E.; Azmi, R.; Li, Z.; Shakouri, S.; Chen, Z.; Zhao-Karger, Z.; Klyatskaya, S.; Maibach, J.; Ruben, M.; Fichtner, M.
2021. ChemSusChem, 14 (8), 1840–1846. doi:10.1002/cssc.202100340Na₃V₂(PO₄)₃ - A Highly Promising Anode and Cathode Material for Sodium-Ion Batteries
Akçay, T.; Häringer, M.; Pfeifer, K.; Anhalt, J.; Binder, J. R.; Dsoke, S.; Kramer, D.; Mönig, R.
2021. ACS applied energy materials, 4 (11), 12688–12695. doi:10.1021/acsaem.1c02413Molecular Vanadium Oxides for Energy Conversion and Energy Storage: Current Trends and Emerging Opportunities
Anjass, M.; Lowe, G. A.; Streb, C.
2021. Angewandte Chemie / International edition, 60 (14), 7522–7532. doi:10.1002/anie.202010577A Brief review of supercapacitors as a novel energy storage device
Bahmei, F.; Bahramifar, N.; Ghasemi, S.; Younesi, H.; Weil, M.
2021. Fuel, ElsevierComparative patent analysis for the identification of global research trends for the case of battery storage, hydrogen and bioenergy
Baumann, M.; Domnik, T.; Haase, M.; Wulf, C.; Emmerich, P.; Rösch, C.; Zapp, P.; Naegler, T.; Weil, M.
2021. Technological forecasting and social change, 165, Art.-Nr.: 120505. doi:10.1016/j.techfore.2020.120505Prospective Life Cycle Assessment of a Model Magnesium Battery
Bautista, S. P.; Weil, M.; Baumann, M.; Tomasini Montenegro, C.
2021. Energy technology, 9 (4), Art.-Nr. 2000964. doi:10.1002/ente.202000964Density Functional Theory Studies on Sulfur-Polyacrylonitrile as a Cathode Host Material for Lithium-Sulfur Batteries
Bertolini, S.; Jacob, T.
2021. ACS Omega, 6 (14), 9700–9708. doi:10.1021/acsomega.0c06240Sodium Cyclopentadienide as a New Type of Electrolyte for Sodium Batteries
Binder, M.; Mandl, M.; Zaubitzer, S.; Wohlfahrt-Mehrens, M.; Passerini, S.; Böse, O.; Danzer, M. A.; Marinaro, M.
2021. ChemElectroChem, 8 (2), 365–369. doi:10.1002/celc.202001290Electrochemical Modeling of Hierarchically Structured Lithium‐Ion Battery Electrodes
Birkholz, O.; Kamlah, M.
2021. Energy technology, 9 (6), Art.-Nr.: 2000910. doi:10.1002/ente.202000910Theoretical studies on the initial oxidation of metallic lithium anodes
Borg, M. van den; Gaissmaier, D.; Knobbe, E.; Fantauzzi, D.; Jacob, T.
2021. Applied Surface Science, 555, Art.-Nr.: 149447. doi:10.1016/j.apsusc.2021.149447Mitigating self-discharge and improving the performance of Mg–S battery in Mg[B(hfip) ] electrolyte with a protective interlayer
Bosubabu, D.; Li, Z.; Meng, Z.; Wang, L.-P.; Fichtner, M.; Zhao-Karger, Z.
2021. Journal of materials chemistry / A, 9 (44), 25150–25159. doi:10.1039/D1TA06114CKadi4Mat : A Research Data Infrastructure for Materials Science
Brandt, N.; Griem, L.; Herrmann, C.; Schoof, E.; Tosato, G.; Zhao, Y.; Zschumme, P.; Selzer, M.
2021. Data science journal, 20 (1), Art.-Nr.: 8. doi:10.5334/dsj-2021-008UHV preparation and electrochemical/-catalytic properties of well-defined Co– and Fe-containing unary and binary oxide model cathodes for the oxygen reduction and oxygen evolution reaction in Zn-air batteries
Buchner, F.; Fuchs, S.; Behm, R. J.
2021. Journal of electroanalytical chemistry, 896, 115497. doi:10.1016/j.jelechem.2021.115497The potential of scanning electrochemical probe microscopy and scanning droplet cells in battery research
Daboss, S.; Rahmanian, F.; Stein, H. S.; Kranz, C.
2021. Electrochemical Science Advances, 2 (4), e2100122. doi:10.1002/elsa.202100122Multiphase-field modeling of spinodal decomposition during intercalation in an Allen-Cahn framework
Daubner, S.; Kubendran Amos, P. G.; Schoof, E.; Santoki, J.; Schneider, D.; Nestler, B.
2021. Physical review materials, 5 (3), Article no: 035406. doi:10.1103/PhysRevMaterials.5.035406On the Electrochemical Insertion of Mg2+in Na7V4(P2O7)4(PO4) and Na3V2(PO4)3 Host Materials
Dongmo, S.; Maroni, F.; Gauckler, C.; Marinaro, M.; Wohlfahrt-Mehrens, M.
2021. Journal of the Electrochemical Society, 168 (12), Art. Nr.: 120541. doi:10.1149/1945-7111/ac412bModeling of Electron‐Transfer Kinetics in Magnesium Electrolytes: Influence of the Solvent on the Battery Performance
Drews, J.; Jankowski, P.; Häcker, J.; Li, Z.; Danner, T.; García Lastra, J. M.; Vegge, T.; Wagner, N.; Friedrich, K. A.; Zhao-Karger, Z.; Fichtner, M.; Latz, A.
2021. ChemSusChem, 14 (21), 4820–4835. doi:10.1002/cssc.202101498An affordable option to Au single crystals through cathodic corrosion of a wire: Fabrication, electrochemical behavior, and applications in electrocatalysis and spectroscopy
Elnagar, M. M.; Hermann, J. M.; Jacob, T.; Kibler, L. A.
2021. Electrochimica acta, 372, 137867. doi:10.1016/j.electacta.2021.137867Energy Flow Analysis of Laboratory Scale Lithium-Ion Battery Cell Production
Erakca, M.; Baumann, M.; Bauer, W.; Biasi, L. de; Hofmann, J.; Bold, B.; Weil, M.
2021. iScience, 24 (5), Article: 102437. doi:10.1016/j.isci.2021.102437Model Studies on the Formation of the Solid Electrolyte Interphase: Reaction of Li with Ultrathin Adsorbed Ionic-Liquid Films and Co O (111) Thin Films
Forster-Tonigold, K.; Kim, J.; Bansmann, J.; Groß, A.; Buchner, F.
2021. ChemPhysChem, 22 (5), 441–454. doi:10.1002/cphc.202001033In operando study of orthorhombic V₂O₅ as positive electrode materials for K-ion batteries
Fu, Q.; Sarapulova, A.; Zhu, L.; Melinte, G.; Missyul, A.; Welter, E.; Luo, X.; Knapp, M.; Ehrenberg, H.; Dsoke, S.
2021. Journal of Energy Chemistry, 62, 627–636. doi:10.1016/j.jechem.2021.04.027Electrochemical performance and reaction mechanism investigation of V₂O₅ positive electrode material for aqueous rechargeable zinc batteries
Fu, Q.; Wang, J.; Sarapulova, A.; Zhu, L.; Missyul, A.; Welter, E.; Luo, X.; Ding, Z.; Knapp, M.; Ehrenberg, H.; Dsoke, S.
2021. Journal of materials chemistry / A, 9 (31), 16776–16786. doi:10.1039/D1TA03518ESupramolecular assembly of a hierarchically structured 3D potassium vanadate framework
Greiner, S.; Anjass, M.; Streb, C.
2021. CrystEngComm, 23 (22), 3946–3950. doi:10.1039/d1ce00661dIron-based perovskites-reduced graphene oxide as possible cathode materials for rechargeable iron-ion battery
Hassan, H. K.; Galal, A.; Atta, N. F.; Jacob, T.
2021. Journal of Alloys and Compounds, 870, Art.-Nr.: 159383. doi:10.1016/j.jallcom.2021.159383Accelerated Kinetics Revealing Metastable Pathways of Magnesiation-Induced Transformations in MnO Polymorphs
Hatakeyama, T.; Li, H.; Okamoto, N. L.; Shimokawa, K.; Kawaguchi, T.; Tanimura, H.; Imashuku, S.; Fichtner, M.; Ichitsubo, T.
2021. Chemistry of Materials, 33 (17), 6983–6996. doi:10.1021/acs.chemmater.1c02011Multiphase-field model for surface diffusion and attachment kinetics in the grand-potential framework
Hoffrogge, P. W.; Mukherjee, A.; Nani, E. S.; Amos, P. G. K.; Wang, F.; Schneider, D.; Nestler, B.
2021. Physical review / E, 103 (3), Article no: 033307. doi:10.1103/PhysRevE.103.033307Comprehensive characterization of propylene carbonate based liquid electrolyte mixtures for sodium-ion cells
Hofmann, A.; Wang, Z.; Bautista, S. P.; Weil, M.; Müller, F.; Löwe, R.; Schneider, L.; Mohsin, I. U.; Hanemann, T.
2021. Electrochimica acta, 403, Art.Nr.: 139670. doi:10.1016/j.electacta.2021.139670Investigation of Parameters Influencing the Producibility of Anodes for Sodium-Ion Battery Cells
Hofmann, J.; Wurba, A.-K.; Bold, B.; Maliha, S.; Schollmeyer, P.; Fleischer, J.; Klemens, J.; Scharfer, P.; Schabel, W.
2021. Production at the leading edge of technology – Proceedings of the 10th Congress of the German Academic Association for Production Technology (WGP), Dresden, 23-24 September 2020. Ed.: B.-A. Behrens, 171–181, Springer. doi:10.1007/978-3-662-62138-7_18Polyoxometalate Modified Separator for Performance Enhancement of Magnesium–Sulfur Batteries
Ji, Y.; Liu-Théato, X.; Xiu, Y.; Indris, S.; Njel, C.; Maibach, J.; Ehrenberg, H.; Fichtner, M.; Zhao-Karger, Z.
2021. Advanced Functional Materials, 31 (26), Art.-Nr.: 2100868. doi:10.1002/adfm.202100868Online adaptive quantum characterization of a nuclear spin
Joas, T.; Schmitt, S.; Santagati, R.; Gentile, A. A.; Bonato, C.; Laing, A.; McGuinness, L. P.; Jelezko, F.
2021. npj Quantum information, 7 (1), 56. doi:10.1038/s41534-021-00389-zPerformance Study of MXene/Carbon Nanotube Composites for Current Collector‐ and Binder‐Free Mg–S Batteries
Kaland, H.; Håskjold Fagerli, F.; Hadler-Jacobsen, J.; Zhao-Karger, Z.; Fichtner, M.; Wiik, K.; Wagner, N. P.
2021. ChemSusChem, 14 (8), 1864–1873. doi:10.1002/cssc.202100173Recent developments and future perspectives of anionic batteries
Karkera, G.; Reddy, M. A.; Fichtner, M.
2021. Journal of power sources, 481, Art.-Nr. 228877. doi:10.1016/j.jpowsour.2020.228877Establishing a Stable Anode–Electrolyte Interface in Mg Batteries by Electrolyte Additive
Li, Z.; Diemant, T.; Meng, Z.; Xiu, Y.; Reupert, A.; Wang, L.; Fichtner, M.; Zhao-Karger, Z.
2021. ACS applied materials & interfaces, 13 (28), 33123–33132. doi:10.1021/acsami.1c08476Simulating mechanical wave propagation within the framework of phase-field modelling
Liu, X.; Schneider, D.; Daubner, S.; Nestler, B.
2021. Computer methods in applied mechanics and engineering, 381, Article: 113842. doi:10.1016/j.cma.2021.113842Self-Standing, Collector-Free Maricite NaFePO4 / Carbon Nanofiber Cathode Endowed with Increasing Electrochemical Activity
Liu-Théato, X.; Indris, S.; Hua, W.; Li, H.; Knapp, M.; Melinte, G.; Ehrenberg, H.
2021. Energy & fuels, 35 (22), 18768–18777. doi:10.1021/acs.energyfuels.1c02779Poly(ionic liquid) Based Composite Electrolytes for Lithium Ion Batteries
Löwe, R.; Hanemann, T.; Zinkevich, T.; Hofmann, A.
2021. Polymers, 13 (24), Article no: 4469. doi:10.3390/polym13244469An Alternative Charge-Storage Mechanism for High-Performance Sodium-Ion and Potassium-Ion Anodes
Ma, Y.; Ma, Y.; Euchner, H.; Liu, X.; Zhang, H.; Qin, B.; Geiger, D.; Biskupek, J.; Carlsson, A.; Kaiser, U.; Groß, A.; Indris, S.; Passerini, S.; Bresser, D.
2021. ACS Energy Letters, 6 (3), 915–924. doi:10.1021/acsenergylett.0c02365Perspective on ultramicroporous carbon as sulphur host for Li–S batteries
Maria Joseph, H.; Fichtner, M.; Munnangi, A. R.
2021. Journal of Energy Chemistry, 59, 242–256. doi:10.1016/j.jechem.2020.11.001Through the Maze of Multivalent‐ion Batteries: A Critical Review on the status of the research on cathode materials for Mg2+ and Ca2+ ions insertion
Maroni, F.; Dongmo, S.; Gauckler, C.; Marinaro, M.; Wolfahrt-Mehrens, M.
2021. Batteries & supercaps, 4 (8), 1221–1251. doi:10.1002/batt.202000330Surface Engineering of a Mg Electrode via a New Additive to Reduce Overpotential
Meng, Z.; Li, Z.; Wang, L.; Diemant, T.; Bosubabu, D.; Tang, Y.; Berthelot, R.; Zhao-Karger, Z.; Fichtner, M.
2021. ACS applied materials & interfaces, 13 (31), 37044–37051. doi:10.1021/acsami.1c07648Comprehensive Electrochemical, Calorimetric Heat Generation and Safety Analysis of Na MnO Cathode Material in Coin Cells
Mohsin, I. U.; Ziebert, C.; Rohde, M.; Seifert, H. J.
2021. Journal of the Electrochemical Society, 168 (5), Art.-Nr. 050544. doi:10.1149/1945-7111/ac0176Thermophysical Characterization of a Layered P2 Type Structure Na₀.₅₃MnO₂Cathode Material for Sodium Ion Batteries
Mohsin, I. U.; Ziebert, C.; Rohde, M.; Seifert, H. J.
2021. Batteries, 7 (1), Article no: 16. doi:10.3390/batteries7010016The metamorphosis of rechargeable magnesium batteries
Mohtadi, R.; Tutusaus, O.; Arthur, T. S.; Zhao-Karger, Z.; Fichtner, M.
2021. Joule, 5 (3), 581–617. doi:10.1016/j.joule.2020.12.021Synthesis and characterization of Ca(₁₋ₓ)SmₓF(₂₊ₓ) (0 ≤ x ≤ 0.15) solid electrolytes for fluoride-ion batteries
Molaiyan, P.; Witter, R.
2021. Material design & processing communications, 3 (5), Art.Nr. e226. doi:10.1002/mdp2.226Structural evolution of a PtRu catalyst in the oxidation of an organic molecule
Mueller, J. E.; Hoffmannová, H.; Hiratoko, T.; Krtil, P.; Jacob, T.
2021. Journal of Catalysis, 398, 89–101. doi:10.1016/j.jcat.2021.04.001Co TiOPO @C as new negative electrode for sodium ion batteries: Synthesis, characterization, and elucidation of the electrochemical mechanism using in operando synchrotron diffraction
Nassiri, A.; Sabi, N.; Sarapulova, A.; Indris, S.; Mangold, S.; Ehrenberg, H.; Saadoune, I.
2021. Journal of Power Sources, 498, Art.-Nr.: 229924. doi:10.1016/j.jpowsour.2021.229924Structure-Property Relation of Trimethyl Ammonium Ionic Liquids for Battery Applications
Rauber, D.; Hofmann, A.; Philippi, F.; Kay, C. W. M.; Zinkevich, T.; Hanemann, T.; Hempelmann, R.
2021. Applied Sciences, 11 (12), 5679. doi:10.3390/app11125679Phase-field formulation of a fictitious domain method for particulate flows interacting with complex and evolving geometries
Reder, M.; Schneider, D.; Wang, F.; Daubner, S.; Nestler, B.
2021. International Journal for Numerical Methods in Fluids, 93 (8), 2486–2507. doi:10.1002/fld.4984Degradation Effects in Metal-Sulfur Batteries
Richter, R.; Häcker, J.; Zhao-Karger, Z.; Danner, T.; Wagner, N.; Fichtner, M.; Friedrich, K. A.; Latz, A.
2021. ACS Applied Energy Materials, 4 (3), 2365–2376. doi:10.1021/acsaem.0c02888Ionic and Thermal Transport in Na-Ion-Conducting Ceramic Electrolytes
Rohde, M.; Mohsin, I. U. I.; Ziebert, C.; Seifert, H. J.
2021. International journal of thermophysics, 42 (10), Art.-Nr.: 136. doi:10.1007/s10765-021-02886-xInvestigation of “Na Co Ti O ” as a multi-phase positive electrode material for sodium batteries
Sabi, N.; Sarapulova, A.; Indris, S.; Dsoke, S.; Trouillet, V.; Mereacre, L.; Ehrenberg, H.; Saadoune, I.
2021. Journal of power sources, 481, Article: 229120. doi:10.1016/j.jpowsour.2020.229120Effect of tortuosity, porosity, and particle size on phase-separation dynamics of ellipsoid-like particles of porous electrodes: Cahn-Hilliard-type phase-field simulations
Santoki, J.; Daubner, S.; Schneider, D.; Kamlah, M.; Nestler, B.
2021. Modelling and simulation in materials science and engineering, 29 (6), Art.Nr. 065010. doi:10.1088/1361-651X/ac11bcEffect of conductivity on the electromigration-induced morphological evolution of islands with high symmetries of surface diffusional anisotropy
Santoki, J.; Mukherjee, A.; Schneider, D.; Nestler, B.
2021. Journal of applied physics, 129 (2), Ar. Nr.: 025110. doi:10.1063/5.0033228Mechanism of Magnesium Transport in Spinel Chalcogenides
Sotoudeh, M.; Dillenz, M.; Groß, A.
2021. Advanced Energy and Sustainability Research, 2 (12), Article no: 2100113. doi:10.1002/aesr.202100113Phase-sensitive quantum spectroscopy with high-frequency resolution
Staudenmaier, N.; Schmitt, S.; McGuinness, L. P.; Jelezko, F.
2021. Physical Review A, 104 (2), Art.-Nr.: L020602. doi:10.1103/PhysRevA.104.L020602High Entropy and Low Symmetry: Triclinic High-Entropy Molybdates
Stenzel, D.; Issac, I.; Wang, K.; Azmi, R.; Singh, R.; Jeong, J.; Najib, S.; Bhattacharya, S. S.; Hahn, H.; Brezesinski, T.; Schweidler, S.; Breitung, B.
2021. Inorganic chemistry, 60 (1), 115–123. doi:10.1021/acs.inorgchem.0c02501ZnS nanoparticles embedded in N-doped porous carbon xerogel as electrode materials for sodium-ion batteries
Tian, G.; Song, Y.; Luo, X.; Zhao, Z.; Han, F.; Chen, J.; Huang, H.; Tang, N.; Dsoke, S.
2021. Journal of alloys and compounds, 877, Art.-Nr.: 160299. doi:10.1016/j.jallcom.2021.160299Environmental assessment of a new generation battery: The magnesium-sulfur system
Tomasini Montenegro, C.; Peters, J. F.; Baumann, M.; Zhao-Karger, Z.; Wolter, C.; Weil, M.
2021. Journal of energy storage, 35, 102053. doi:10.1016/j.est.2020.102053Preparation of intergrown P/O-type biphasic layered oxides as high-performance cathodes for sodium ion batteries
Wang, K.; Wu, Z.-G.; Melinte, G.; Yang, Z.-G.; Sarkar, A.; Hua, W.; Mu, X.; Yin, Z.-W.; Li, J.-T.; Guo, X.-D.; Zhong, B.-H.; Kübel, C.
2021. Journal of Materials Chemistry A, 9 (22), 13151–13160. doi:10.1039/d1ta00627dInfluence of Complexing Additives on the Reversible Deposition/Dissolution of Magnesium in an Ionic Liquid
Weber, I.; Ingenmey, J.; Schnaidt, J.; Kirchner, B.; Behm, R. J.
2021. ChemElectroChem, 8 (2), 390–402. doi:10.1002/celc.202001488Batteries Europe - Task Force SUSTAINABILITY POSITION PAPER
Wiesner, E.; Bardé, F.; Weil, M.; Borbujo, Y. C.; Edström, K.; Kiuru, J.; Rizo-Martin, J.; Metz, P. de; Pettit, C.; Poliscanova, J.; Ramon, N. G.; Santos, C.
2021. Sustainability Task ForceNanodiamond Theranostic for Light-Controlled Intracellular Heating and Nanoscale Temperature Sensing
Wu, Y.; Alam, M. N. A.; Balasubramanian, P.; Ermakova, A.; Fischer, S.; Barth, H.; Wagner, M.; Raabe, M.; Jelezko, F.; Weil, T.
2021. Nano letters, 21 (9), 3780–3788. doi:10.1021/acs.nanolett.1c00043Enhanced Potassium Storage Capability of Two-Dimensional Transition-Metal Chalcogenides Enabled by a Collective Strategy
Wu, Y.; Zhang, Q.; Xu, Y.; Xu, R.; Li, L.; Li, Y.; Zhang, C.; Zhao, H.; Wang, S.; Kaiser, U.; Lei, Y.
2021. ACS applied materials & interfaces, 13 (16), 18838–18848. doi:10.1021/acsami.1c01891Microstructure evolution and intermediate phase-induced varying solubility limits and stress reduction behavior in sodium ion batteries particles of NaᵪFePO (0< <1 )ᵪ
Zhang, T.; Kamlah, M.
2021. Journal of power sources, 483, Article: 229187. doi:10.1016/j.jpowsour.2020.229187 -
2020Nanoscale Dynamic Readout of a Chemical Redox Process Using Radicals Coupled with Nitrogen-Vacancy Centers in Nanodiamonds
Barton, J.; Gulka, M.; Tarabek, J.; Mindarava, Y.; Wang, Z.; Schimer, J.; Raabova, H.; Bednar, J.; Plenio, M. B.; Jelezko, F.; Nesladek, M.; Cigler, P.
2020. ACS Nano, 14 (10), 12938–12950. doi:10.1021/acsnano.0c04010Exploratory multi-criteria decision analysis of utility-scale battery storage technologies for multiple grid services based on life cycle approaches
Baumann, M.; Peters, J.; Weil, M.
2020. Energy technology, 8 (11), Art.Nr. 1901019. doi:10.1002/ente.201901019ORR and OER on Ni-modified Co3O4(111) Cathodes for Zn-Air Batteries - A Combined Surface Science and Electrochemical Model Study
Behm, R. J.; Buchner, F.; Eckardt, M.; Böhler, T.; Kim, J.; Gerlach, J.; Schnaidt, J.
2020. ChemSusChem, 13 (12), 3199–3211. doi:10.1002/cssc.202000503Investigation on the formation of Mg metal anode/electrolyte interfaces in Mg/S batteries with electrolyte additives
Bhaghavathi Parambath, V.; Zhao-Karger, Z.; Diemant, T.; Jäckle, M.; Li, Z.; Scherer, T.; Gross, A.; Behm, R. J.; Fichtner, M.
2020. Journal of materials chemistry / A, 8 (43), 22998–23010. doi:10.1039/d0ta05762bExploring the Structure–Activity Relationship on Platinum Nanoparticles
Braunwarth, L.; Jung, C.; Jacob, T.
2020. Topics in catalysis, 63, 1647–1657. doi:10.1007/s11244-020-01324-wScreening of Charge Carrier Migration in the MgSc Se Spinel Structure
Dillenz, M.; Sotoudeh, M.; Euchner, H.; Groß, A.
2020. Frontiers in energy research, 8, Article no: 584654. doi:10.3389/fenrg.2020.584654Stripping and Plating a Magnesium Metal Anode in Bromide‐Based Non‐Nucleophilic Electrolytes
Dongmo, S.; Zaubitzer, S.; Schüler, P.; Krieck, S.; Jörissen, L.; Wohlfahrt-Mehrens, M.; Westerhausen, M.; Marinaro, M.
2020. ChemSusChem, 13 (13), 3530–3538. doi:10.1002/cssc.202000249Modeling of Ion Agglomeration in Magnesium Electrolytes and its Impacts on Battery Performance
Drews, J.; Danner, T.; Jankowski, P.; Vegge, T.; García Lastra, J. M.; Liu, R.; Zhao-Karger, Z.; Fichtner, M.; Latz, A.
2020. ChemSusChem, 13 (14), 3599–3604. doi:10.1002/cssc.202001034Influence of Additives on the Reversible Oxygen Reduction Reaction/Oxygen Evolution Reaction in the Mg²⁺‐Containing Ionic Liquid N ‐Butyl‐N ‐Methylpyrrolidinium Bis(Trifluoromethanesulfonyl)imide
Eckardt, M.; Alwast, D.; Schnaidt, J.; Behm, R. J.
2020. ChemSusChem, 13 (15), 3919–3927. doi:10.1002/cssc.202000672Controlled-Atmosphere Flame Fusion Growth of Nickel Poly-oriented Spherical Single Crystals—Unraveling Decades of Impossibility
Esau, D.; Schuett, F. M.; Varvaris, K. L.; Björk, J.; Jacob, T.; Jerkiewicz, G.
2020. Electrocatalysis, 11 (1), 1–13. doi:10.1007/s12678-019-00575-wFirst results from in situ transmission electron microscopy studies of all-solid-state fluoride ion batteries
Fawey, M. H.; Chakravadhanula, V. S. K.; Munnangi, A. R.; Rongeat, C.; Hahn, H.; Fichtner, M.; Kübel, C.
2020. Journal of power sources, 466, Article: 228283. doi:10.1016/j.jpowsour.2020.228283Magnesium Batteries: Research and Applications
Fichtner, M.
2020. Royal Society of Chemistry (RSC). doi:10.1039/9781788016407Microscopic Properties of Na and Li—A First Principle Study of Metal Battery Anode Materials
Gaissmaier, D.; Borg, M.; Fantauzzi, D.; Jacob, T.
2020. ChemSusChem, 13 (4), 771–783. doi:10.1002/cssc.201902860Phase transformation, charge transfer, and ionic diffusion of Na MnV(PO ) in sodium-ion batteries: a combined first-principles and experimental study
Gao, X.; Lian, R.; He, L.; Fu, Q.; Indris, S.; Schwarz, B.; Wang, X.; Chen, G.; Ehrenberg, H.; Wei, Y.
2020. Journal of materials chemistry / A, 8 (34), 17477–17486. doi:10.1039/d0ta05929cDynamics of porous and amorphous magnesium borohydride to understand solid state Mg-ion-conductors
Heere, M.; Hansen, A.-L.; Payandeh, S. H.; Aslan, N.; Gizer, G.; Sørby, M. H.; Hauback, B. C.; Pistidda, C.; Dornheim, M.; Lohstroh, W.
2020. Scientific reports, 10 (1), Article No. 9080. doi:10.1038/s41598-020-65857-6Investigation of N and S Co-doped Porous Carbon for Sodium-Ion Battery, Synthesized by Using Ammonium Sulphate for Simultaneous Activation and Heteroatom Doping
Ikram, S.; Dsoke, S.; Sarapulova, A.; Müller, M.; Rana, U. A.; Siddiqi, H. M.
2020. Journal of the Electrochemical Society, 167 (10), Article: 100531. doi:10.1149/1945-7111/ab9a01Inactive materials matter: How binder amounts affect the cycle life of graphite electrodes in potassium-ion batteries
Jeschull, F.; Maibach, J.
2020. Electrochemistry communications, 121, Art.-Nr. 106874. doi:10.1016/j.elecom.2020.106874Properties and Structural Arrangements of the Electrode Material CuDEPP during Energy Storage
Jung, C. K.; Stottmeister, D.; Jacob, T.
2020. Energy technology, 8 (9), Art.Nr. 2000388. doi:10.1002/ente.202000388Environmental Sustainability Assessment of Multi-Sectoral Energy Transformation Pathways: Methodological Approach and Case Study for Germany
Junne, T.; Simon, S.; Buchgeister, J.; Saiger, M.; Baumann, M.; Haase, M.; Wulf, C.; Naegler, T.
2020. Sustainability, 12 (19), Art.-Nr. 8225. doi:10.3390/su12198225Interaction between Li, Ultrathin Adsorbed Ethylene Carbonate Films, and CoO(111) Thin Films: A Model Study of the Solid Electrolyte Interphase Formation at CoO Anodes
Kim, J.; Buchner, F.; Behm, R. J.
2020. The journal of physical chemistry <Washington, DC> / C, 124 (39), 21476–21490. doi:10.1021/acs.jpcc.0c06015Rechargeable Calcium–Sulfur Batteries Enabled by an Efficient Borate-Based Electrolyte
Li, Z.; Vinayan, B. P.; Diemant, T.; Behm, R. J.; Fichtner, M.; Zhao-Karger, Z.
2020. Small, 16 (39), Art.-Nr.: 2001806. doi:10.1002/smll.202001806Multi‐Electron Reactions enabled by Anion‐Based Redox Chemistry for High‐Energy Multivalent Rechargeable Batteries
Li, Z.; Vinayan, B. P.; Jankowski, P.; Njel, C.; Roy, A.; Vegge, T.; Maibach, J.; Lastra, J. M. G.; Fichtner, M.; Zhao-Karger, Z.
2020. Angewandte Chemie / International edition, 59 (28), 11483–11490. doi:10.1002/anie.202002560Entropy Changes upon Double Layer Charging at a (111)-Textured Au Film in Pure 1-Butyl-1-Methylpyrrolidinium Bis[(trifluoromethyl)sulfonyl]imide Ionic Liquid
Lindner, J.; Weick, F.; Endres, F.; Schuster, R.
2020. The journal of physical chemistry <Washington, DC> / C, 124 (1), 693–700. doi:10.1021/acs.jpcc.9b09871In situ Observation of Sodium Dendrite Growth and Concurrent Mechanical Property Measurements Using an Environmental Transmission Electron Microscopy–Atomic Force Microscopy (ETEM-AFM) Platform
Liu, Q.; Zhang, L.; Sun, H.; Geng, L.; Li, Y.; Tang, Y.; Jia, P.; Wang, Z.; Dai, Q.; Shen, T.; Tang, Y.; Zhu, T.; Huang, J.
2020. ACS energy letters, 5 (8), 2546–2559. doi:10.1021/acsenergylett.0c01214A 3d-printed composite electrode for sustained electrocatalytic oxygen evolution
Liu, S.; Liu, R.; Gao, D.; Trentin, I.; Streb, C.
2020. Chemical communications, 56 (60), 8476–8479. doi:10.1039/D0CC03579CCopper Porphyrin as a Stable Cathode for High‐Performance Rechargeable Potassium Organic Batteries
Lv, S.; Yuan, J.; Chen, Z.; Gao, P.; Shu, H.; Yang, X.; Liu, E.; Tan, S.; Ruben, M.; Zhao-Karger, Z.; Fichtner, M.
2020. ChemSusChem, 13 (9), 2286–2294. doi:10.1002/cssc.202000425Molecular Scylla and Charybdis: Maneuvering between pH Sensitivity and Excited-State Localization in Ruthenium Bi(benz)imidazole Complexes
Mengele, A. K.; Müller, C.; Nauroozi, D.; Kupfer, S.; Dietzek, B.; Rau, S.
2020. Inorganic chemistry, 59 (17), 12097–12110. doi:10.1021/acs.inorgchem.0c01022Toward a cell-chemistry specific life cycle assessment of lithium-ion battery recycling processes
Mohr, M.; Peters, J. F.; Baumann, M.; Weil, M.
2020. Journal of industrial ecology, 24 (6), 1310–1322. doi:10.1111/jiec.13021Electrochemical Performance of Carbon Modified LiNiPO as Li-Ion Battery Cathode: A Combined Experimental and Theoretical Study
Nasir, M. H.; Janjua, N. K.; Santoki, J.
2020. Journal of the Electrochemical Society, 167 (13), 130526. doi:10.1149/1945-7111/abb83dBenchmark for Synthesized Diamond Sensors Based on Isotopically Engineered Nitrogen‐Vacancy Spin Ensembles for Magnetometry Applications
Osterkamp, C.; Balasubramanian, P.; Wolff, G.; Teraji, T.; Nesladek, M.; Jelezko, F.
2020. Advanced Quantum Technologies, 3 (9), Art.-Nr.: 2000074. doi:10.1002/qute.202000074Understanding the mechanism of byproduct formation within operandosynchrotron techniques and its effects on the electrochemical performance of VO (B) nanoflakes in aqueous rechargeable zinc batteries
Pang, Q.; Zhao, H.; Lian, R.; Fu, Q.; Wei, Y.; Sarapulova, A.; Sun, J.; Wang, C.; Chen, G.; Ehrenberg, H.
2020. Journal of materials chemistry / A, 8 (19), 9567–9578. doi:10.1039/d0ta00858cNew maximally disordered – High entropy intermetallic phases (MD-HEIP) of the Gd La Sn Sb M (M=Li, Na, Mg): Synthesis, structure and some properties
Pavlyuk, V.; Balińska, A.; Rożdżyńska-Kiełbik, B.; Pavlyuk, N.; Dmytriv, G.; Stetskiv, A.; Indris, S.; Schwarz, B.; Ehrenberg, H.
2020. Journal of alloys and compounds, 838, Art. Nr.: 155643. doi:10.1016/j.jallcom.2020.155643Choosing the right carbon additive is of vital importance for high-performance Sb-based Na-ion batteries
Pfeifer, K.; Arnold, S.; Budak, Ö.; Luo, X.; Presser, V.; Ehrenberg, H.; Dsoke, S.
2020. Journal of materials chemistry / A, 2020 (8), 6092–6104. doi:10.1039/D0TA00254BThe Interaction Between Electrolytes and Sb2O3–based Electrodes in Sodium Batteries: Uncovering Detrimental Effects of Diglyme
Pfeifer, K.; Greenstein, M. F.; Aurbach, D.; Luo, X.; Ehrenberg, H.; Dsoke, S.
2020. ChemElectroChem, 7 (16), 3487–3495. doi:10.1002/celc.202000894Controlled‐Atmosphere Flame Fusion Single‐Crystal Growth of Non‐Noble fcc, hcp, and bcc Metals Using Copper, Cobalt, and Iron
Schuett, F. M.; Esau, D.; Varvaris, K. L.; Gelman, S.; Björk, J.; Rosen, J.; Jerkiewicz, G.; Jacob, T.
2020. Angewandte Chemie / International edition, 59 (32), 13246–13252. doi:10.1002/anie.201915389Strain Dependence of Metal Anode Surface Properties
Stottmeister, D.; Groß, A.
2020. ChemSusChem, 13 (12), 3147–3153. doi:10.1002/cssc.202000709Surface Science and Electrochemical Model Studies on the Interaction of Graphite and Li‐Containing Ionic Liquids
Weber, I.; Kim, J.; Buchner, F.; Schnaidt, J.; Behm, R. J.
2020. ChemSusChem, 13 (10), 2589–2601. doi:10.1002/cssc.202000495A digital workflow for learning the reduced-order structure-property linkages for permeability of porous membranes
Yabansu, Y. C.; Altschuh, P.; Hötzer, J.; Selzer, M.; Nestler, B.; Kalidindi, S. R.
2020. Acta materialia, 195, 668–680. doi:10.1016/j.actamat.2020.06.003Probing the Effect of Titanium Substitution on the Sodium Storage in Na₃Ni₂BiO₆ Honeycomb-Type Structure
Zemlyanushin, E.; Pfeifer, K.; Sarapulova, A.; Etter, M.; Ehrenberg, H.; Dsoke, S.
2020. Energies, 13 (24), Article: 6498. doi:10.3390/en13246498Mechanically Coupled Phase-Field Modeling of Microstructure Evolution in Sodium Ion Batteries Particles of NaₓFePO₄
Zhang, T.; Kamlah, M.
2020. Journal of the Electrochemical Society, 167 (2), Art. Nr.: 020508. doi:10.1149/1945-7111/ab645aHeat Generation in NMC622 Coin Cells during Electrochemical Cycling: Separation of Reversible and Irreversible Heat Effects
Zhao, W.; Rohde, M.; Mohsin, I. U.; Ziebert, C.; Seifert, H. J.
2020. Batteries, 6 (4), Article: 55. doi:10.3390/batteries6040055 -
2019Modeling the effective conductivity of the solid and the pore phase in granular materials using resistor networks
Birkholz, O.; Gan, Y.; Kamlah, M.
2019. Powder technology, 351, 54–65. doi:10.1016/j.powtec.2019.04.005Interaction between Li, Ultrathin Adsorbed Ionic Liquid Films, and CoO(111) Thin Films: A Model Study of the Solid|Electrolyte Interphase Formation
Buchner, F.; Forster-Tonigold, K.; Kim, J.; Bansmann, J.; Groß, A.; Behm, R. J.
2019. Chemistry of materials, 31 (15), 5537–5549. doi:10.1021/acs.chemmater.9b01253A Lithium‐Free Energy‐Storage Device Based on an Alkyne‐Substituted‐Porphyrin Complex
Chen, Z.; Gao, P.; Wang, W.; Klyatskaya, S.; Zhao-Karger, Z.; Wang, D.; Kübel, C.; Fuhr, O.; Fichtner, M.; Ruben, M.
2019. ChemSusChem, 12 (16), 3737–3741. doi:10.1002/CSSC.201901541Difference in Electrochemical Mechanism of SnO₂ Conversion in Lithium-Ion and Sodium-Ion Batteries: Combined in Operando and Ex Situ XAS Investigations
Dixon, D.; Ávila, M.; Ehrenberg, H.; Bhaskar, A.
2019. ACS omega, 4 (6), 9731–9738. doi:10.1021/acsomega.9b00563Exploits, advances and challenges benefiting beyond Li-ion battery technologies
El Kharbachi, A.; Zavorotynska, O.; Latroche, M.; Cuevas, F.; Yartys, V.; Fichtner, M.
2019. Journal of alloys and compounds, 817, Article no: 153261. doi:10.1016/j.jallcom.2019.153261Influence of electric fields on metal self-diffusion barriers and its consequences on dendrite growth in batteries
Jäckle, M.; Groß, A.
2019. The journal of chemical physics, 151 (23), Article no: 234707. doi:10.1063/1.5133429Surface chemistry and electrochemistry of an ionic liquid and lithium on Li₄Ti₅O₁₂(111) - A model study of the anode|electrolyte interface
Kim, J.; Weber, I.; Buchner, F.; Schnaidt, J.; Behm, R. J.
2019. The journal of chemical physics, 151 (13), Article no: 134704. doi:10.1063/1.5119765Hetero-layered MoS /C composites enabling ultrafast and durable Na storage
Li, Z.; Liu, S.; Vinayan, B. P.; Zhao-Karger, Z.; Diemant, T.; Wang, K.; Behm, R. J.; Kübel, C.; Klingeler, R.; Fichtner, M.
2019. Energy storage materials, 21, 115–123. doi:10.1016/j.ensm.2019.05.042Towards stable and efficient electrolytes for room-temperature rechargeable calcium batteries
Li, Z.; Fuhr, O.; Fichtner, M.; Zhao-Karger, Z.
2019. Energy & environmental science, 12 (12), 3496–3501. doi:10.1039/c9ee01699fDirect Conversion of CO₂ to Multi-Layer Graphene using Cu–Pd Alloys
Molina-Jirón, C.; Chellali, M. R.; Kumar, C. N. S.; Kübel, C.; Velasco, L.; Hahn, H.; Moreno-Pineda, E.; Ruben, M.
2019. ChemSusChem, 12 (15), 3509–3514. doi:10.1002/cssc.201901404Ni TiOPO phosphate: Sodium insertion mechanism and electrochemical performance in sodium-ion batteries
Nassiri, A.; Sabi, N.; Sarapulova, A.; Dahbi, M.; Indris, S.; Ehrenberg, H.; Saadoune, I.
2019. Journal of power sources, 418, 211–217. doi:10.1016/j.jpowsour.2019.02.038Interface in Solid-State Lithium Battery: Challenges, Progress, and Outlook
Pervez, S. A.; Cambaz, M. A.; Thangadurai, V.; Fichtner, M.
2019. ACS applied materials & interfaces, 11 (25), 22029–22050. doi:10.1021/acsami.9b02675A review of hard carbon anode materials for sodium-ion batteries and their environmental assessment
Peters, J. F.; Abdelbaky, M.; Baumann, M.; Weil, M.
2019. Matériaux & techniques, 107 (5), Article No. 503. doi:10.1051/mattech/2019029Can Metallic Sodium Electrodes Affect the Electrochemistry of Sodium‐Ion Batteries? Reactivity Issues and Perspectives
Pfeifer, K.; Arnold, S.; Becherer, J.; Das, C.; Maibach, J.; Ehrenberg, H.; Dsoke, S.
2019. ChemSusChem, 12 (14), 3312–3319. doi:10.1002/cssc.201901056Electromigration in Lithium Whisker Formation Plays Insignificant Role during Electroplating
Rulev, A. A.; Sergeev, A. V.; Yashina, L. V.; Jacob, T.; Itkis, D. M.
2019. ChemElectroChem, 6 (5), 1324–1328. doi:10.1002/celc.201801652Evidence of a Pseudo-Capacitive Behavior Combined with an Insertion/Extraction Reaction Upon Cycling of the Positive Electrode Material P2-Na x Co 0.9 Ti 0.1 O 2 for Sodium-ion Batteries
Sabi, N.; Sarapulova, A.; Indris, S.; Dsoke, S.; Zhao, Z.; Dahbi, M.; Ehrenberg, H.; Saadoune, I.
2019. ChemElectroChem, 6 (3), 892–903. doi:10.1002/celc.201801870Role of conductivity on the electromigration-induced morphological evolution of inclusions in {110}-oriented single crystal metallic thin films
Santoki, J.; Mukherjee, A.; Schneider, D.; Nestler, B.
2019. Journal of applied physics, 126 (16), Article No.165305. doi:10.1063/1.5119714A quasielastic and inelastic neutron scattering study of the alkaline and alkaline-earth borohydrides LiBH 4 and Mg(BH 4 ) 2 and the mixture LiBH 4 + Mg(BH 4 ) 2
Silvi, L.; Zhao-Karger, Z.; Röhm, E.; Fichtner, M.; Petry, W.; Lohstroh, W.
2019. Physical chemistry, chemical physics, 21 (2), 718–728. doi:10.1039/c8cp04316gInsights into the electrochemical processes of rechargeable magnesium–sulfur batteries with a new cathode design
Vinayan, B. P.; Euchner, H.; Zhao-Karger, Z.; Cambaz, M. A.; Li, Z.; Diemant, T.; Behm, R. J.; Gross, A.; Fichtner, M.
2019. Journal of materials chemistry / A, 7 (44), 25490–25502. doi:10.1039/c9ta09155fMgSc Se - A Magnesium Solid Ionic Conductor for All-Solid-State Mg Batteries?
Wang, L.-P.; Zhao-Karger, Z.; Klein, F.; Chable, J.; Braun, T.; Schür, A. R.; Wang, C.-R.; Guo, Y.-G.; Fichtner, M.
2019. ChemSusChem, 12 (10), 2286–2293. doi:10.1002/cssc.201900225Beyond Intercalation Chemistry for Rechargeable Mg Batteries: A Short Review and Perspective
Zhao-Karger, Z.; Fichtner, M.
2019. Frontiers in Chemistry, 6, Article: 656. doi:10.3389/fchem.2018.00656